Microwave Chemistry: Principles and Applications
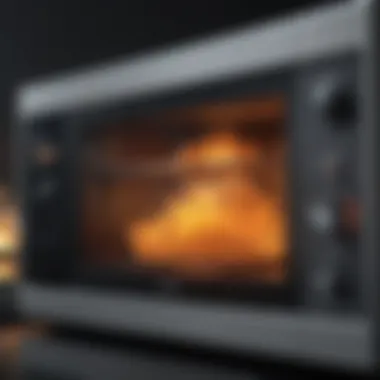
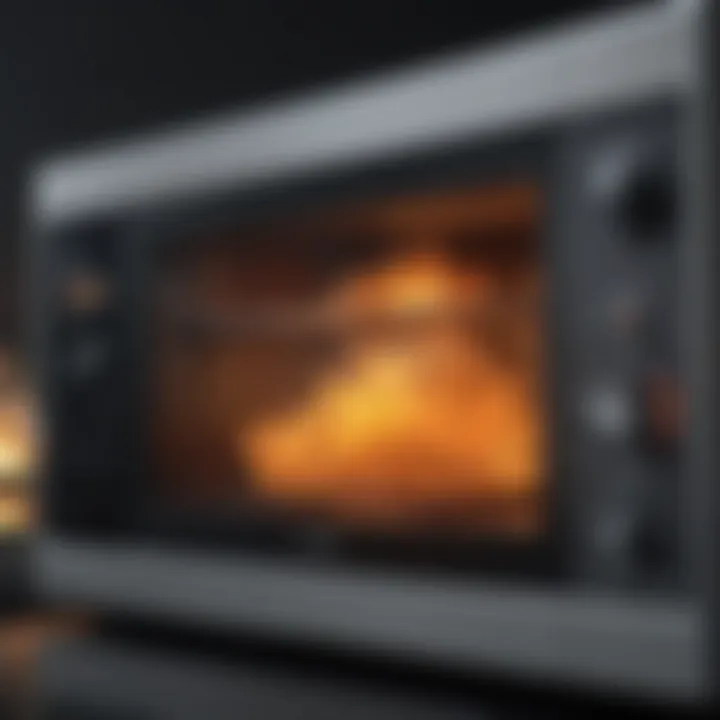
Intro
Microwave chemistry is an increasingly pivotal field that combines the science of microwave energy with chemical processes. It harnesses the unique interaction of microwaves with substances to facilitate and enhance various chemical reactions. Think of it as channeling the power of microwaves, not to heat leftovers, but to unlock new pathways and efficiencies in chemistry.
This section will introduce the fundamental aspects of microwave chemistry, illuminating how it has transformed traditional methods. In the past, chemical reactions required considerable time and careful temperature management, often leading to inconsistent results. Now, researchers can leverage microwave technology to obtain better yields in a fraction of the time.
This transformation is not merely a convenience. It’s a leap in scientific capability, allowing chemists to explore previously uncharted territory in both organic and material chemistry. The unsuspecting microwave on your kitchen counter signifies a breakthrough in laboratory principles, where molecules dance to a rhythm dictated by specific microwave wavelengths.
A key takeaway is that although microwave chemistry has roots in basic physical chemistry, its applications stretch wide and include diverse fields ranging from academia to industrial manufacturing and even pharmaceuticals.
As we venture deeper into the nitty-gritty of this fascinating field, we will discuss its principles and methodologies, the challenges it faces, and the incredible advantages it offers to modern-day researchers.
Prelims to Microwave Chemistry
Microwave chemistry is a fascinating branch of chemical science that harnesses the power of microwaves to facilitate and enhance chemical reactions. The relevance of this subject has steadily grown as researchers and industries alike seek more efficient, eco-friendly approaches to chemical processes. By utilizing microwave energy, reactions can occur faster, often with improved yields. This article aims to delve into the intricate workings of microwave chemistry, highlighting its principles, methodologies, and broad spectrum of applications within organic and synthetic chemistry.
Historical Background and Development
The journey of microwave chemistry began in the mid-20th century when scientists first recognized the potential of microwaves in heating substances. Initially, the use of microwaves was limited, largely confined to cooking. However, as researchers experimented, they discovered that microwaves could do more than just heat food; they could drive chemical reactions to completion more rapidly than traditional methods. The work of scientists like K. C. Nicolaou in the 1990s played a pivotal role in showcasing the advantages of this technology. Through extensive studies, it became increasingly clear that microwave-assisted synthesis could revolutionize how chemists approached reaction mechanisms.
Definition and Scope
Microwave chemistry involves the application of microwave radiation to promote chemical reactions. This technique exploits the dielectric heating effect, which occurs when polar molecules within a substance interact with microwave radiation, leading to rapid heating. The scope of microwave chemistry encompasses various fields, such as organic synthesis, polymer chemistry, and even medicinal chemistry. By providing a more controlled environment and significantly reducing reaction times, microwave chemistry has opened doors for new synthetic routes and innovative applications.
The significance of understanding microwave chemistry cannot be understated, particularly at a time when sustainability and efficiency are crucial. Researchers find that the application of microwave-assisted techniques not only helps in achieving faster reactions but also plays a vital role in reducing solvent usage and advancing greener chemistry.
"Microwave chemistry reflects a paradigm shift in how we think about chemical reactivity and synthesis, enabling scientists to explore uncharted territories with efficiency and precision."
As we proceed through this exploration of microwave chemistry, we will systematically unpack the fundamental principles, the myriad synthesis techniques, and the diverse applications that mark the evolution of this significant science.
Fundamental Principles of Microwave Chemistry
Understanding the fundamental principles of microwave chemistry forms the bedrock of the entire field. This segment delves into how microwaves interact with different materials and the essential dielectric properties at play. Such intricacies are not merely academic but practical, offering significant implications for both research and application.
Microwave Interaction with Matter
Microwave radiation primarily interacts with polar molecules. Think of water; its dipolar nature means it responds very well to microwave energy. When exposed to microwaves, the orientation of water molecules rapidly flips, which generates heat almost instantaneously. This is what allows for the effective application of heat in various chemical reactions without the need for conventional heating.
However, interactions can vary based on the molecular structure of the substances involved. For instance, salts or non-polar materials don't absorb microwaves effectively, hence they usually require a different approach to synthesis or processing. This interaction mechanism can lead to rapid reaction times and better energy efficiencies compared to traditional methods. In practical terms, it allows for more streamlined processes, often reducing reaction times from hours to mere minutes.
Some key points to consider include:
- Efficiency: Microwave-assisted reactions tend to be faster and require less energy.
- Selectivity: Certain reactions may yield purer products due to precise temperature control.
- Safety: Reduced exposure to high temperatures minimizes risks associated with volatile reactions.
"Microwave chemistry transforms how we approach synthesis, giving us unprecedented control over reaction conditions."
Dielectric Properties of Substances
A fundamental understanding of dielectric properties is crucial in microwave chemistry. These properties essentially describe how a substance responds to an external electric field. Different materials exhibit various dielectric constants, which influence how well they can absorb microwave energy.
For instance, in solvents, the dielectric constant can determine whether the microwave will penetrate effectively or just skim the surface. High dielectric constant materials can absorb microwaves efficiently, leading to quicker energy transformation to thermal energy. Conversely, low dielectric substances often require additional additives or co-solvents to facilitate effective microwave absorption.
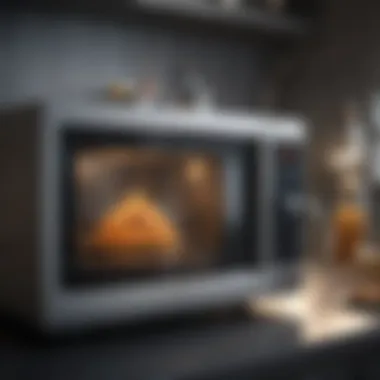
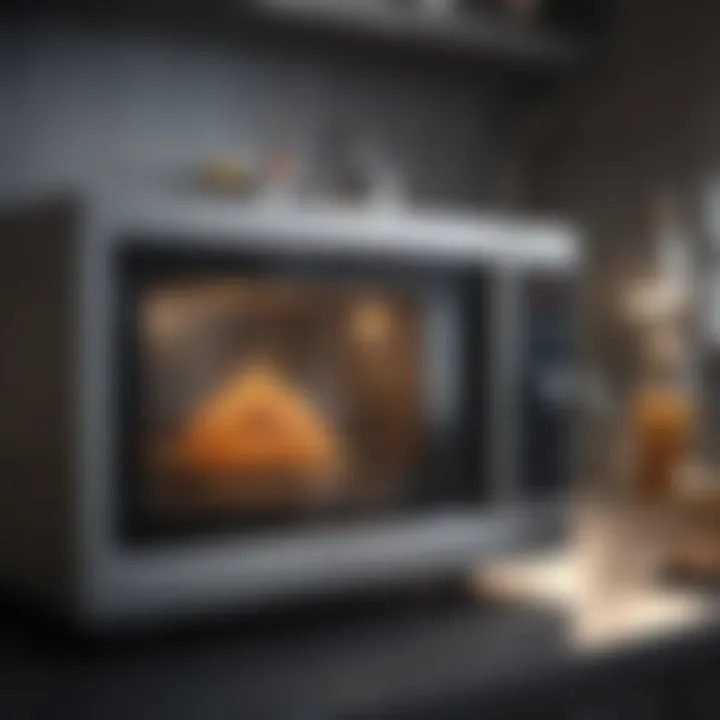
This concept is particularly relevant in optimizing reactions:
- Polar solvents, such as dimethyl sulfoxide (DMSO) or ethylene glycol, often enhance reaction rates thanks to their favorable dielectric properties.
- Solid supports used in microwave-assisted synthesis, such as silica or polymer-based materials, are often selected based on their dielectric profiles.
In summary, a nuanced grasp of how microwaves interact with various substances and their dielectric properties is pivotal. It lays the groundwork for understanding why microwave-assisted techniques are increasingly favored in modern chemistry. Integrating these principles into practical applications can lead to advancements that save time, energy, and resources.
Microwave-assisted Synthesis Techniques
Microwave-assisted synthesis techniques have carved a niche in modern chemistry, offering a powerful alternative to traditional methods. This approach leverages microwave radiation to hasten chemical reactions, leading to enhanced efficiencies in various synthesis processes. By aptly controlling temperature and pressure, researchers can tackle problems that would otherwise require extensive resources and time. This section dives into specifics like solid-phase microwave reactions and microwave-induced organic reactions, both of which have proven to be notable facets in achieving better outcomes in chemical research.
Solid-phase Microwave Reactions
Solid-phase microwave reactions involve the use of microwave energy to facilitate chemical transformations within solid supports. This method has gained traction, especially in organic synthesis and material science. The advantages are tangible: rapid heating, minimal solvent usage, and the potential for high throughput in reaction screening.
- Efficiency: Unlike conventional heating methods that expose all components of the mixture uniformly and slowly, microwaves selectively heat the solid phase. This can significantly decrease reaction times.
- Reproducibility: As solid-phase methodologies often involve fixed matrices, they allow for better control over reaction variables. This uniformity leads to higher reproducibility and refinement of product formation.
- Versatility: Solid-phase techniques can be adapted to various contexts such as peptide synthesis, where solid supports become essential;
- Resource Savings: Since many reactions can be conducted in an environmentally friendly manner with little to no solvents, these solid-phase techniques align well with the growing push for sustainability in chemistry.
- For instance, polystyrene or resin-based systems are quite popular in this regard.
To highlight the significance of this technique, a recent study showcased a potential ingenuity in peptide synthesis using solid-phase microwave reactions, dramatically reducing synthesis time without sacrificing yield. This clearly illustrates the transformative nature of microwave-assisted techniques.
"Solid-phase microwave reactions not only accelerate the process but also allow for greater precision in controlling how reactions unfold."
Microwave-induced Organic Reactions
Microwave-induced organic reactions represent another significant stride in the field of microwave chemistry. At the heart of this approach is the utilization of microwave energy to enhance organic reactions, often yielding higher conversions and selectivity. These reactions can vary widely from cycloadditions to coupling reactions.
- Rapid Reaction Times: One of the main benefits of this method is how quickly reactions can progress. In many instances, a reaction that might have taken hours or even days can be completed in minutes.
- Higher Yields: Microwave conditions often lead to fewer by-products — the energy input optimizes conditions for the desired reaction pathway
- Solvent-Free Reactions: Many microwave-induced organic reactions have been demonstrated in solvent-free conditions, which supports both greener chemistry initiatives and reduces the need for costly solvents.
- Scalable Techniques: While numerous studies center on small-scale applications, the principles behind microwave-induced organic reactions can be translated into larger setups, making them immensely practical for industrial applications as well.
Examples worth noting include the use of microwave technology in the synthesis of biologically significant compounds like indole derivatives or the efficient preparation of heterocycles, further showcasing the nuances of this technology. These innovations show how this approach continues to drive the frontiers of organic chemistry, reshaping methodologies and enhancing accessibility.
Applications of Microwave Chemistry
The realm of microwave chemistry has transformed how we approach various chemical disciplines. Its significance is not just in the techniques employed, but in the efficiency and effectiveness it brings to a multitude of applications. By harnessing the properties of microwaves, scientists are achieving results that were once thought impossible within reasonable time frames and under lower energy inputs. Let's delve deeper into the specific applications that showcase the importance of this method.
Synthetic Organic Chemistry
In synthetic organic chemistry, microwaves are heralding a new age of reaction conditions. Traditionally, reactions often required long durations, with heat sources that could lead to overheating or uneven heating. With microwave-assisted synthesis, reactions happen rapidly due to uniform heating and direct energy absorption by the reactants. This not only shortens reaction times significantly but also opens the door to unique reaction pathways.
Consider a typical scenario: a chemist aims to synthesize a complex organic molecule that, via conventional methods, may take several hours or even days. Using microwave technology, this could be achieved in mere minutes, often leading to higher yields and purity of the products.
Some key benefits include:
- Greater selectivity for desired products due to controlled heating.
- Reduced solvent use, aiding in greener chemistry.
- Enhanced reproducibility, crucial for scaling laboratory methods to industrial applications.
Polymer Chemistry
The application of microwave technology in polymer chemistry is not just a figment of imagination; it’s very much a reality reshaping the field. By using microwaves, scientists can accelerate polymerization reactions, leading to the formation of materials with superior properties. In many cases, the properties of the polymer can be tailored simply by adjusting the heating conditions provided by microwaves.
For instance, emulsion polymerization, a common method for producing polymers, can be conducted in a fraction of the time compared to conventional methods. This rapid processing reduces the energy needed and aids in producing finer materials that can be employed in coatings, adhesives, and various other applications.
Some notable points:
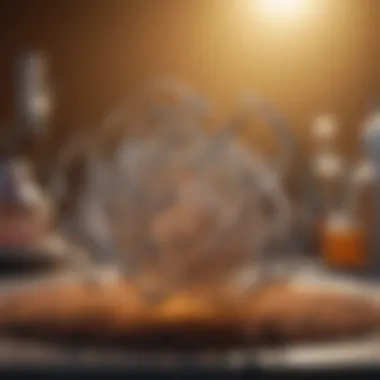
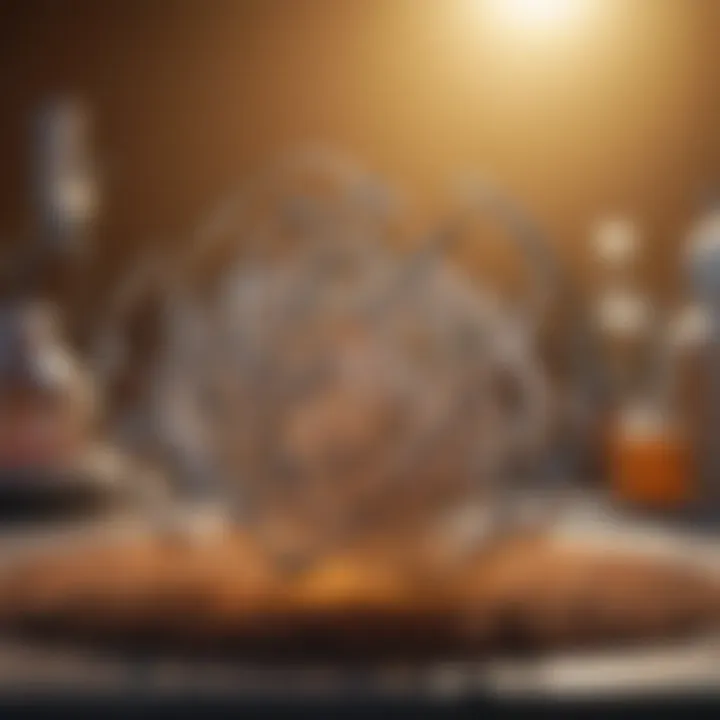
- They often create nanoscale materials that have exceptional characteristics compared to their bulk counterparts.
- Control over the molecular weight distribution, allowing for the design of specialty polymers for specific applications.
Inorganic Synthesis
Microwave chemistry is equally adept when it comes to inorganic synthesis. With the ability to provide precise heating, this method enables the synthesis of diverse inorganic materials such as nanoparticles, metal oxides, and coordination complexes. The kinetic advantages observed in reactions are particularly useful in producing compounds that are otherwise challenging to synthesize through conventional heating methods.
Reactions such as sol-gel processes and the synthesis of solid-state materials benefit greatly from microwave techniques, often resulting in materials with better structural integrity and purity. Among the distinct attributes:
- Fast synthesis of nanomaterials with controlled sizes and morphologies.
- Increased reaction rates leading to fewer byproducts and higher yields.
Biochemistry and Pharmaceutical Applications
The implications of microwave chemistry extend profoundly into biochemistry and pharmaceuticals. In drug discovery, the efficiency of microwave-assisted synthesis can lead to the rapid generation of libraries of compounds for high-throughput screening. This capability can drastically enhance the speed of identifying potential drug candidates, vital in today’s fast-paced pharmaceutical landscape.
Moreover, microwave technology is applied in biocatalysis, optimizing reaction conditions to improve the production of bioactive compounds. This trend indicates a shift toward more sustainable practices in pharmaceutical manufacturing. Key highlights of its impact in this area include:
- The ability to synthesize complex biomolecules in shorter timeframes.
- Enhancements in reaction conditions, minimizing the degradation of sensitive compounds.
"The application of microwave technology in the pharmaceutical industry is like turning the dial back on inefficiencies, making way for a brighter and more productive future."
Advantages of Microwave Technology in Chemistry
Microwave technology has started to carve out a significant niche within the realm of chemical synthesis, offering several advantages that are not only noteworthy but transformative. Its importance is underscored by its ability to change the pace at which reactions occur, leading to more efficient and effective synthetic processes. As we delve into the nuanced benefits of this technology, we will explore how these advantages stack up against traditional methodologies, enhancing overall research and application in chemistry.
Enhanced Reaction Rates
One of the cornerstones of microwave chemistry is the substantially increased reaction rates it can achieve. By harnessing the unique interacting properties of microwaves with materials, the reaction environments are effectively optimized. Microwaves penetrate the reaction medium, delivering energy uniformly and rapidly, which can induce reactions to proceed faster than conventionally heated methods. In practice, this can mean reducing reaction times from hours to mere minutes.
For example, consider a reaction that typically requires heating on a stovetop for several hours. A microwave reaction may complete in a fraction of that time, allowing chemists to explore a wider range of conditions or reactions in a single workday. This capability drastically increases the throughput of material synthesis, making research labs far more productive and advancing the pace of discovery.
Improved Yield and Selectivity
Another significant advantage of using microwave techniques lies in the enhanced yield and selectivity of products. This is attributable to the precise control over reaction conditions afforded by microwave irradiation. By adjusting factors such as the power and time of exposure, researchers can fine-tune reactions to preferentially produce desired products while minimizing by-products. This is critical in any synthesis, especially when economic or ecological concerns push for high purity and low waste.
In certain organic reactions, using a microwave has been shown to not only boost yield but also improve the selectivity of the products formed. For instance, in coupling reactions typical in organic synthesis, the use of microwave technology can lead to cleaner reactions with fewer undesired side-products. This increased efficiency is not just beneficial in terms of output but also aids in further stages of processing, such as purification.
Environmentally Friendly Protocols
The push towards greener chemistry has become a significant narrative in recent decades. Microwave technology conveniently aligns with this ethos by promoting environmentally friendly synthesis protocols. Traditional heating often requires large amounts of solvents and valuable reagents, leading to increased waste and energy consumption. However, microwave-assisted reactions often employ solvent-free methods or significantly reduce the amount of solvent needed while effectively enhancing reaction yields.
A compelling example is the synthesis of biodiesel where microwave-assisted transesterification reduces by-products and reaction time, thus making the entire process more sustainable. Moreover, since microwave reactions can lead to lower energy usage due to shorter reaction times, this not only stands to benefit the environment but also the overall costs associated with chemical manufacturing.
"Utilizing microwave technology in chemistry can push the boundaries of modern synthesis, making processes faster, greener, and more efficient."
Overall, as we examine the advantages of microwave technology, it becomes apparent that its application is a major step forward in the quest for enhanced chemical synthesis. The combination of faster reaction rates, improved yield and selectivity, alongside environmentally sound practices, makes microwave chemistry an increasingly attractive option for researchers and professionals alike.
Challenges and Limitations
Microwave chemistry has made significant strides in enhancing chemical synthesis, yet it's not without its hurdles. Understanding these challenges is fundamental, not only to harness its full potential but also to ensure safe and efficient practices in laboratory settings and industrial applications. The balance between innovation and practicality often dictates the success and acceptance of microwave-assisted techniques among chemists and researchers. Several elements must be considered when delving into the difficulties associated with this microwave-driven approach.
Equipment and Safety Concerns
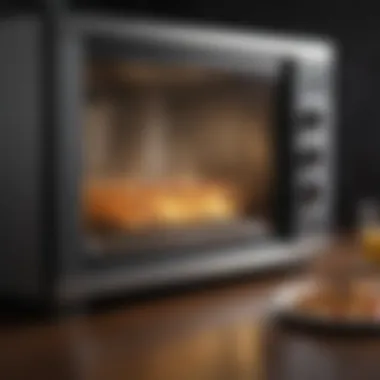
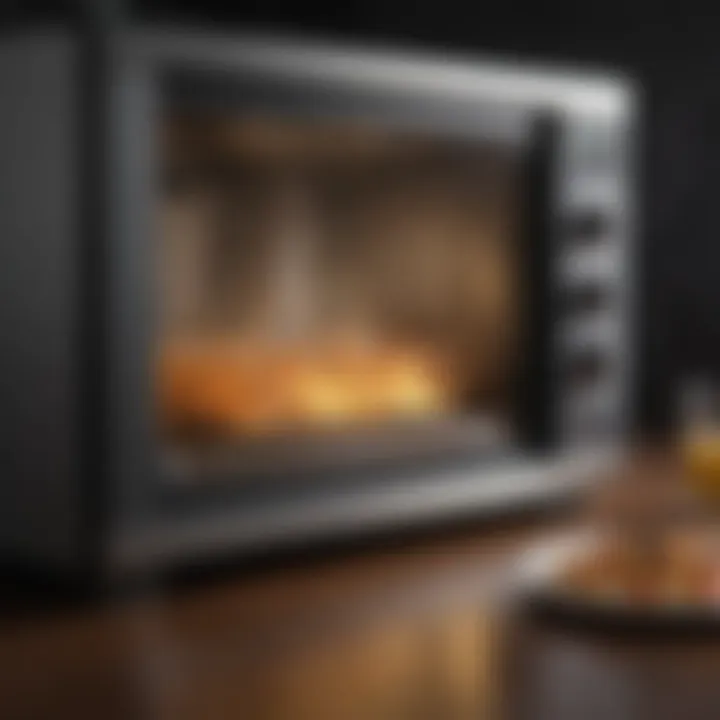
One prominent challenge is the equipment and safety issues related to the use of microwave technology in chemical processes. Consider, for instance, the specifics of the microwave reactors. These specialized instruments require careful thought as they differ markedly from conventional heating mediums. The design must accommodate certain parameters, such as pressure and temperature, which can significantly influence reaction outcomes.
Microwave equipment can be expensive and might not always deliver uniform heating, leading to hotspots that could compromise the integrity of sensitive reactions. Additionally, when working with hazardous substances, the risk of explosions or burns rises with increased temperatures and pressures. This fact mandates strict safety protocols and thorough training for users. The implementation of proper shielding, automatic cut-offs, and reliable monitoring systems is crucial for minimizing risks.
Moreover, laboratory layouts must be reconsidered. These systems often need dedicated spaces that meet specific ventilation and safety standards, thus potentially increasing installation costs.
"Safety is not just the absence of danger; it is the presence of proper safeguards."
Scalability Issues
Scalability is another critical aspect that poses limitations for broad applications of microwave chemistry. Although studies in small-scale systems often yield excellent results, translating these methods to larger scales remains fraught with complications. The reproducibility of results tends to falter as the volume increases, creating disparities in yields and product qualities. Moreover, what works in a test tube might not necessarily perform well in an industrial setting where variables become more complex.
Key considerations include:
- Heating Uniformity: In larger volumes, maintaining consistent heating becomes a formidable obstacle. While microwaves can penetrate substances, larger batches can absorb energy unevenly, leading to hot and cold spots.
- Reaction Time: The efficiency scales differently. Long reaction times that are manageable in lab experiments may become impractical in commercial production.
- Economic Feasibility: The cost of materials and energy for scaling up often doesn’t align with the expected profit margins, making large-scale microwave synthesis challenging to adopt.
Future of Microwave Chemistry
As we stand on the cusp of technological breakthroughs in chemistry, microwave chemistry is poised to play an integral role. This field of study, rooted in the principles of rapid heating and effective molecular interaction, holds promise for extensive applications in various sectors. From enhancing reaction efficiencies to introducing entirely new synthetic pathways, the future is likely bright. Understanding these advancements not only appeals to the chemical industrialist but also ignites curiosity in students and researchers alike.
The importance of microwave chemistry lies in its potential to transform existing methodologies. With growing concerns over environmental sustainability and the need for cost-effective solutions, the efficiency of microwave-mediated processes cannot be overlooked.
One significant aspect of this future is the development of greener protocols. The reduction of solvents in chemical processes is a hot topic in recent years. Microwave applications promise a decrease in chemical waste while maintaining high yields. More so, the ability to accelerate reactions under mild conditions results in lower energy consumption, which comes as a welcome relief to both chemists and environmentalists.
"The future remains in innovation and adaptation, and microwave chemistry offers a front-row seat to both."
Emerging Trends and Innovations
The emergence of novel materials and catalysts through microwave-assisted techniques is indeed remarkable. For instance, the synthesis of nanomaterials has shown considerable advancements. Techniques such as microwave-assisted hydrothermal synthesis streamline the creation of these materials, yielding uniform particles with enhanced properties. As a result, applications in electronics and biomedical fields are starting to gain foothold.
Moreover, the advent of programmable microwave systems allows chemists to tailor specific reaction conditions. This adaptability can unlock new avenues in organic synthesis and pharmaceutics. A recent highlight includes the use of microwave technology in peptide synthesis, pushing the boundaries of what's feasible in pharmaceutical research.
The potential for machine learning in optimizing microwave reactions is another compelling trend. With data-driven approaches, chemists can predict outcomes more accurately, resulting in faster development cycles and better resource allocation. The integration of artificial intelligence with microwave chemistry could indeed represent a monumental shift for the field.
Integration with Other Technologies
The synergy between microwave technology and other scientific innovations opens a myriad of possibilities. One noteworthy aspect is the combination of microwave-assisted synthesis with 3D printing. This blending may enable the rapid production of complex structures with bespoke materials, allowing fields like biomedical engineering and materials science to flourish.
Additionally, biotechnology stands to gain significantly from the integration of microwave chemistry. The rapid DNA amplification techniques paired with microwave heating demonstrate enhanced efficiency, possibly revolutionizing molecular biology research.
**Conclusion: The future of microwave chemistry, filled with trends and the potential for integration, paints an optimistic picture for various scientific disciplines. As students, researchers, and professionals reflect on these advancements, the importance of adapting and evolving within the realm of microwave technologies becomes increasingly clear. While challenges remain, the prospects encourage a forward-thinking attitude, propelling us into a new era of chemistry.
End
Microwave chemistry offers a compelling glimpse into the future of chemical synthesis and material science. It stands out not just for its novelty, but for the profound efficiencies it can inject into traditional methods of chemical reactions. From accelerating reaction times to improving yields, the advancements in this field demonstrate significant potential, particularly in contexts where speed and efficiency matter immensely.
Summary of Key Points
- Enhanced Reaction Rates: Microwave-assisted processes can drastically reduce the time required for reactions to occur. This efficiency can open doors for rapid development in various chemical applications, reducing overall project timelines.
- Environmental Considerations: The methods associated with microwave technology often favor greener chemistry practices, aligning with contemporary sustainability goals in research.
- Diverse Applications: From organic chemistry to biochemistry, the versatility of microwave chemistry means it can be harnessed across a spectrum of disciplines, paving the way for innovative solutions in drug development and materials engineering.
- Challenges to Overcome: While the advantages are numerous, limitations such as equipment costs and scalability concerns must be acknowledged. Understanding these challenges is necessary for further advancements in microwave chemistry.
- Future Trajectories: The integration of microwave techniques with other advanced technologies holds exciting possibilities. Innovations might reshape how researchers engage with chemistry in years to come.
Final Thoughts on Microwave Chemistry
Microwave chemistry is not just a passing trend; it's reshaping our approach to chemical research and applications. As scientists continue to delve into this area, the potential for novel discoveries and improved methodologies remains vast. Embracing this technology may well yield not only better efficiencies but also foster a deeper understanding of chemical processes at a molecular level.
In essence, the future looks bright for microwave chemistry, and its influence is likely to expand as researchers continue to explore and refine its applications.
Embracing microwave chemistry may enhance the landscape of chemical research by unlocking faster, more efficient synthesis methods.