Implications and Applications of Genome Sequencing Depth
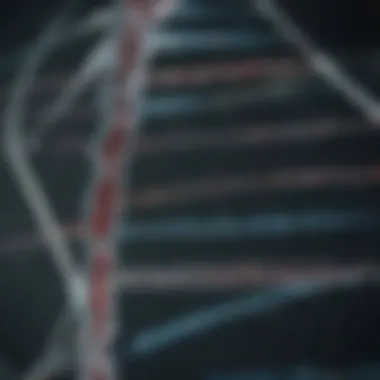
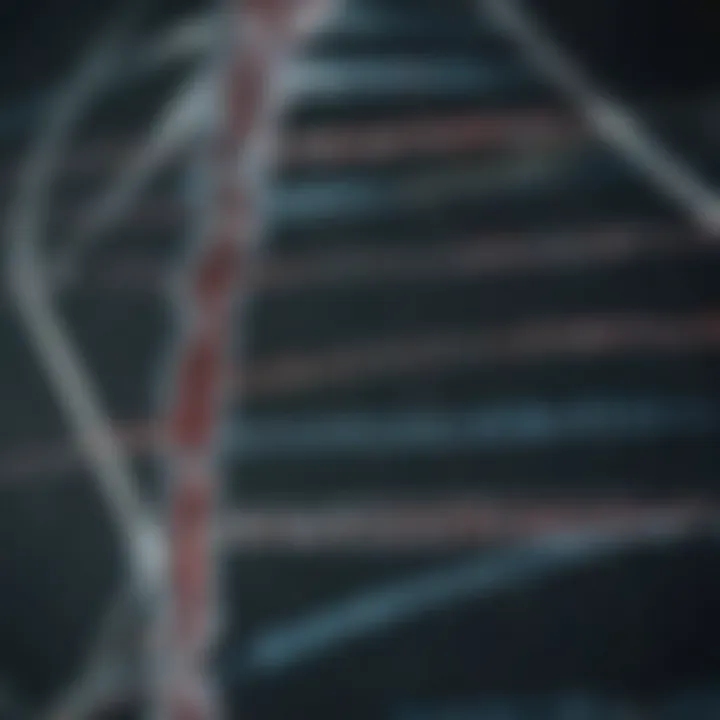
Intro
Whole genome sequencing (WGS) has taken the genomic world by storm, acting as a beacon for researchers in fields ranging from medical science to evolutionary biology. At the heart of this innovation lies the concept of sequencing depth, a term that embodies the number of times a specific segment of the genome is read during the sequencing process. It's not merely a technical specification; it's a critical determinant of the quality and reliability of genomic data. As scientists push the envelope of what WGS can achieve, understanding the implications of sequencing depth becomes imperative for impeccable research outcomes and clinical applications.
The advent of WGS has presented a golden opportunity for dissecting complex genomic structures, identifying genetic variations, and understanding the underpinnings of diseases. Yet, the efficacy of these advances hinges on achieving optimal sequencing depth. This section unpacks key findings and sets the stage for a deeper discussion on the implications and applications of WGS depth across various scientific domains.
Understanding Whole Genome Sequencing
Whole genome sequencing is a powerful tool that allows researchers to dive into the full genetic makeup of an organism. Understanding this topic forms the foundation for unraveling mysteries in genetics, opening up avenues in various fields like medicine, microbiology, and evolutionary studies. As we move deeper into the age of personalized medicine, the insights drawn from whole genome sequencing will become increasingly vital.
The importance of grasping the principles of whole genome sequencing lies not only in technical aspects but also in its implications. This method can provide illuminating clues about genetic predispositions to diseases, which can lead to more targeted treatments. For example, understanding the intricacies of a patientās genome can help in choosing the right medication, reducing adverse effects, and increasing treatment efficacy.
Moreover, whole genome sequencing has significant applications in studying complex genetic disorders. The data derived can help researchers identify variations that contribute to certain diseases, influencing both therapeutic approaches and public health strategies.
Definition and Overview
In simplest terms, whole genome sequencing (WGS) is the process of determining the complete DNA sequence of an organism's genome. This approach captures insights from both coding and non-coding regions of the genome, allowing for a comprehensive analysis. Unlike targeted gene sequencing, which focuses on specific genes, WGS offers a panoramic view of genetic material.
WGS employs various methodologies to analyze the DNA. The result is a wealth of information on genetic variations and potential markers linked to diseases. This method has shifted the paradigm in genomics, offering unparalleled resolution that can aid not just basic research but also clinical applications.
Historical Context
The journey of whole genome sequencing began in the late 20th century, but its significance surged with the completion of the Human Genome Project in 2003. This monumental task sequenced the human genome and laid the groundwork for future genomic studies. The implications of such comprehensive data are enormous; it not only unlocked secrets of human biology but also set the stage for advancements in medical research.
Initially, sequencing was fraught with challenges, primarily due to high costs and the limitations of available technologies. Lineage from the early Sanger sequencing methods to next-generation sequencing (NGS) technologies represents a significant leap. NGS has made the process more efficient and accessible, so much so that today, whole genome sequencing can be performed at a fraction of the initial cost.
Whole genome sequencing transformed the landscape of genomics, turning once-imposing tasks into achievable goals for researchers across the globe.
In the years following, innovations and refined techniques have perpetually enhanced our understanding of genetics. The rise of computational biology, bioinformatics, and advanced analytical techniques has complemented WGS, leading to powerful insights and actionable data in fields such as oncology, cardiology, and pharmacogenomics. Each step forward adds layers of complexityāand opportunityāto the science of genetics.
What is Sequencing Depth?
In the realm of genomics, sequencing depth is a term that echoes with significance. Understanding this concept is essential for grasping how comprehensive and accurate a genomic analysis can be. Sequencing depth, also known as coverage, refers to the number of times a particular base in the genome is read during sequencing. In simpler terms, itās like having several versions of a book, where each versionāeach readāprovides different insights. The depth of sequence coverage not only influences the reliability of results but also impacts the feasibility of various applications across diverse fields, including medicine and biology.
Conceptual Framework
To break it down further, sequencing depth can be thought of as the backbone of genomic data quality. A higher depth means more reads, enhancing the likelihood of correctly identifying variants, particularly those present in low abundance. But what does this mean in practical terms? Imagine a library; the more copies of a book you have, the easier it is to spot errors or nuances. In genomic sequencing, the implications are profound. Higher sequencing depths allow researchers to detect subtle variations within the DNA that might hold critical information for understanding diseases, evolutionary changes, or microbial diversity.
However, itās also crucial to consider diminishing returns. Beyond a certain point, increasing the depth offers little improvement in variant detection and can lead to unnecessary expenses without yielding proportionate benefits. For instance, in a clinical context, if the cost of sequencing is significantly ramped-up without tangible benefits in diagnostic accuracy, it raises questions about resource allocation and feasibility.
Measurement Techniques
Measuring sequencing depth is no walk in the park. It necessitates a variety of techniques ranging from computational algorithms to laboratory practices. Most commonly, depth is quantified using the formula:
This equation allows researchers to calculate the average coverage across the genome or specific regions of interest. But thereās more to the story. There are several measurement techniques that researchers rely on:
- Base Calling: Algorithms analyze the sequence data to determine the nucleotide sequence.
- Mapping to Reference Genomes: Reads are aligned to known reference genomes to assess how many times each region of the genome is covered.
- Read Depth Statistical Analysis: Researchers often utilize statistical models to assess uniformity and bias in coverage levels.
These techniques highlight not only the numerical aspect of sequencing depth but also the complexities involved in analyzing the vast genomic data collected.
Understanding sequencing depth is pivotal for harnessing the full potential of genomic studies. The importance lies in how it shapes research, drives innovative applications in clinical settings, and influences the overall quality of genomic information. As this field continues to evolve, so too will the methods of measuring and applying sequencing depth in ways that enhance our understanding of biology and health.
The Importance of Sequencing Depth
Sequencing depth is like the foundation of a house in the realm of genomic analysis. It essentially affects everything that comes after it, from the quality of the data to the reliability of conclusions drawn. This section brings to light why understanding and optimizing sequencing depth is of utmost importance in various applications, such as clinical diagnostics and evolutionary studies.
Enhancing Data Quality
When it comes to genomics, data quality is non-negotiable. High sequencing depth can significantly reduce the chances of errors that arise from low coverage. Consider a library where each book represents a sequence of DNA. If you only read a few pages of each book, youāre likely to miss vital information, right? Likewise, a higher sequencing depth allows researchers to cross-verify sequences, making sure that what they read isnāt just a fluke but a consistent reading of the genetic material. Consequently, this enhances the overall reliability of the genomic data collected. Itās especially crucial when dealing with complex genomes that carry significant variation across individuals.
Impact on Variant Detection
The ability to detect genetic variants accurately defines the success of genomic studies. Variants like single nucleotide variants and structural variants carry information that can illuminate inherited traits, predispositions to certain diseases, and more. Thus, itās vital to understand how depth influences this detection process.
Single Nucleotide Variants
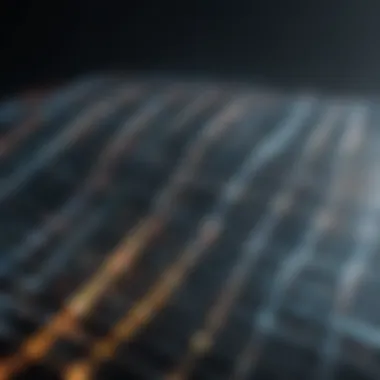
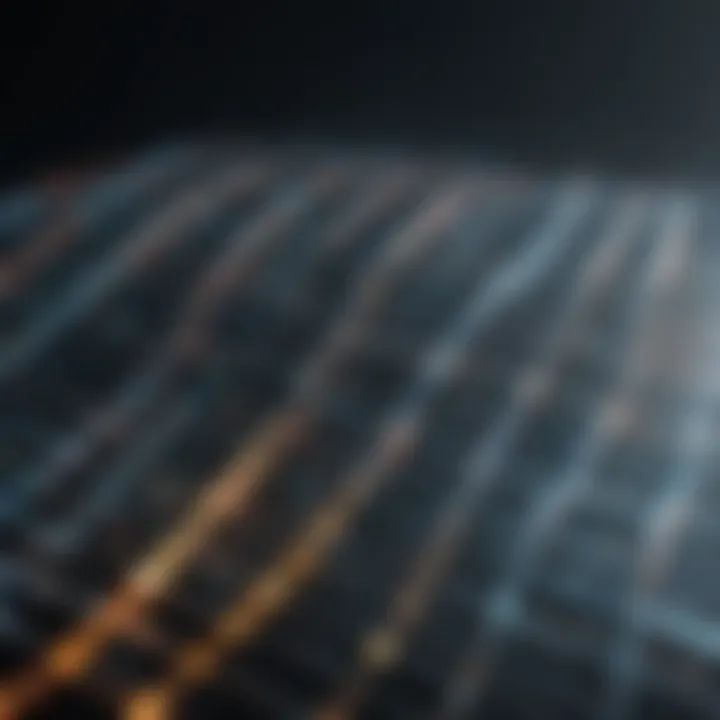
Single nucleotide variants, often dubbed SNVs, play a major role in genetic diversity. These tiny changes can have profound implications on gene function. A key characteristic of SNVs is that they are relatively straightforward to detect, especially when sequencing depth is substantial. When depth is high, it lowers the noise to signal ratio, allowing these subtle changes to emerge with clarity. This makes SNVs a favorite among researchers because they provide a wealth of information with relative ease.
However, relying solely on SNVs might not paint the entire picture. For example, low-frequency variants can slip under the radar if depth isnāt adequately accounted for. So, while SNVs offer a treasure trove of insights, researchers must complement them with other methods to gain a comprehensive view of genetic variation.
Structural Variants
On the flip side, structural variants are more experimental and less straightforward than their single-nucleotide counterparts. Structural variants include larger-scale changes in the genome such as deletions, duplications, and inversions. Their complexity can skew results if sequencing depth is not sufficient. One unique feature of structural variants is their potential involvement in significant phenotypic differences.
For instance, in certain cancers, structural variants might lead to altered gene expression leading to tumorigenesis. However, because these variants are larger and require a more nuanced approach for detection, insufficient depth can lead to missed calls. Therefore, while they are essential for understanding complex traits and diseases, ensuring adequate sequencing depth is imperative to catch them reliably.
In summary, sequencing depth acts as a crucial player in optimizing the detection of variants, significantly impacting the quality of genetic analysis. Itās important for researchers to balance depth to capture both SNVs and structural variants effectively, adapting their strategies based on the specific needs of their studies.
Factors Influencing Sequencing Depth
Understanding the factors that influence sequencing depth is crucial for optimizing whole genome sequencing projects. These factors can significantly affect the outcomes regarding data quality, the ability to detect variants, and the overall interpretation of genomic information. By addressing these elements, researchers can improve their methodologies and achieve more reliable and comprehensive analyses.
Library Preparation
Library preparation is a fundamental step that shapes the sequencing depth achieved in a study. It begins with extracting DNA from the sample, which can differ based on the source, such as blood, saliva, or tissues. A clean and efficient extraction ensures that enough high-quality DNA is available for subsequent processing.
Once the DNA is obtained, it must be fragmented and ligated to adapters that will allow it to be sequenced. The efficiency of this process can vary greatly and is often influenced by the quality and concentration of starting material. An appropriate balance must be struck; too much DNA can lead to excessive complexity, while too little can result in missed insights.
In summary, meticulous library preparation directly correlates with sequencing depth by influencing the quantity and quality of DNA available for sequencing, ultimately leading to more accurate results.
Sequencing Technology
The technology employed for sequencing profoundly impacts how sequencing depth is achieved. Over the years, several platforms have emerged, each offering distinct advantages and challenges. Below are three major players in the sequencing arena:
Illumina Platforms
Illumina sequencing technology has become a cornerstone in genomics due to its high-throughput capabilities and cost-effectiveness. One key characteristic of Illumina platforms is their ability to generate millions of short reads in parallel. This feature significantly increases the throughput while keeping costs comparatively low.
However, Illumina's focus on short-read sequencing poses a challenge. It can sometimes lead to difficulties in resolving complex genomic regions, especially in areas with repetitive sequences. Nonetheless, for many applications, like clinical genomics and RNA sequencing, the accuracy and comprehensiveness it offers make it an invaluable choice.
Pacific Biosciences
Pacific Biosciences, often referred to as PacBio, stands out for its long-read sequencing capabilities. These reads provide in-depth insights into structural variations and complex regions of the genome. The power of PacBio lies in its real-time sequencing technology, allowing researchers to capture longer stretches of DNA without the need for complex library preparation.
One major drawback, though, is the cost associated with running PacBio sequencers, which can be significantly higher than traditional Illumina platforms. However, its strength lies in applications where long reads are essential, such as de novo genome assembly or the study of complex genomic regions that short reads might miss.
Oxford Nanopore Technologies
Oxford Nanopore Technologies offers a unique approach to sequencing using nanopore technology allowing for real-time analysis of long DNA strands. This is particularly advantageous for applications needing quick turnaround times, as it permits immediate data analysis as the DNA strands pass through the nanopores.
A notable benefit of Oxford Nanopore is the portability of its devices, making it possible to collect genomic data in varied settings, from laboratories to field studies. However, the overall accuracy of the sequencing can sometimes lag behind other platforms, presenting a trade-off between speed and precision.
"Choosing the right sequencing technology is akin to picking a tool for a job; it largely depends on the specific requirements and constraints of your project."
Each technology, therefore, presents both advantages and limitations that influence sequencing depth and the quality of genomic insights derived from the study. Researchers must consider these factors carefully when designing their approaches to maximize the benefits of whole genome sequencing.
Optimal Sequencing Depth: Standards and Recommendations
Sequencing depth is not just a technical specification; it represents a critical benchmark in genomic research that influences data accuracy and utility. Setting optimal standards for sequencing depth is paramount because it dictates the reliability of findings in various applications ranging from clinical diagnostics to evolutionary studies. Understanding how to navigate these depths allows researchers and clinicians to extract meaningful insights from genetic material while keeping costs manageable.
Recommended Depth for Different Applications
Clinical Genomics
Clinical genomics focuses on the diagnosis and treatment of diseases based on genomic information. When it comes to sequencing depth, typically 30x is often recommended for the detection of a range of variants, including those linked to hereditary conditions.
The key characteristic of clinical genomics is its direct impact on patient management and care, often resulting in tailored therapeutic approaches. This is a beneficial aspect, as personalized medicine can lead to improved outcomes. One unique feature of clinical genomics is the necessity for accuracy - while deeper sequencing can lead to more variant detection, itās crucial that the data remains interpretable and subject to clinical validation. However, the main disadvantage lies in the accumulated costs associated with higher depth and the potential for diminishing returns past a certain point.
Population Genomics
Population genomics aims to understand genetic variation and its correlation with evolutionary processes across different populations. In this area, a sequencing depth of around 10x to 15x is often adequate for exploring common variations and studying population structures.
One key characteristic of population genomics is its focus on broader genetic trends rather than individual variants. Itās a popular choice because understanding variation on this scale can elucidate human history and health disparities. A unique feature here is the ability to detect rare alleles, which could be missed at shallower depths. The disadvantage, however, is that while deeper sequencing improves the detection of rare variants, it may not always be economically justified, especially in large-scale projects with tight budgets.
Microbial Genomics
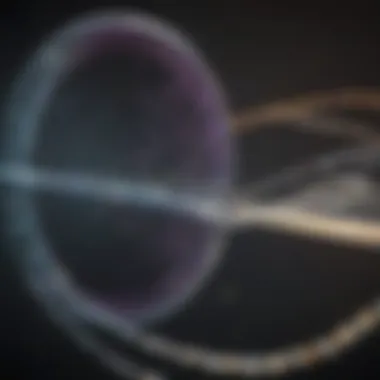
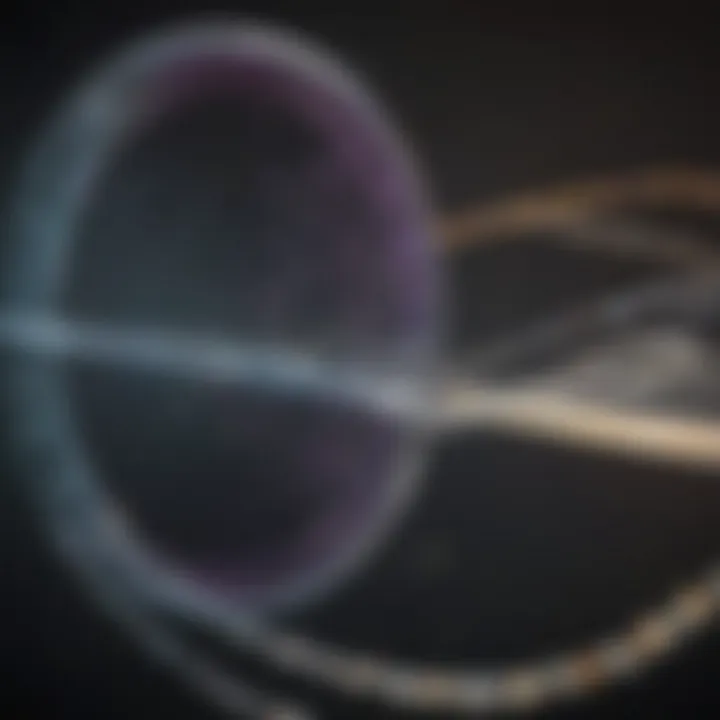
Microbial genomics deals with the study of microorganisms and their genetic material. A recommended depth of 50x or higher is often suggested for comprehensive strain-level resolution.
A distinctive characteristic of microbial genomics is its applications in areas like public health and antibiotic resistance. This makes it a beneficial instrument for tracking outbreaks and evolutionary trends in pathogens. Microbial genomes are often more complex, and higher depth sequencing captures the full taxonomy and variation within a sample effectively. However, one notable disadvantage is the potential for extensive data that can overwhelm existing analytical frameworks and necessitate advanced computational resources.
Balancing Cost and Efficiency
Balancing the costs associated with sequencing depth against the efficiency of obtaining reliable data is an essential task for researchers. It requires not just an assessment of financial inputs, but also a clear alignment with the goals of the research. As depths increase, costs can spiral quickly, and results may yield marginal benefits.
Optimal sequencing depth doesn't follow a one-size-fits-all model. Factors including the specific objectives of the study, the nuances of the sample types, and inherent biological variability all create a complex landscape for decision-making. In order to achieve a balance, some strategies may include:
- Strategic Targeting: Focusing depth on regions of interest rather than the entire genome can help manage costs effectively.
- Phased Approaches: Performing initial sequencing at a base depth with targeted follow-up at greater depth for specific variants of interest can be economically viable.
- Pooling Samples: To enhance cost-effectiveness, pooling multiple samples together for sequencing can reduce expenses while still providing valuable insights.
Ultimately, understanding the standards and recommendations for optimal sequencing depth is about making informed, strategic decisions that maximize the quality and relevance of genomic data.
Challenges in Achieving Optimal Depth
Achieving the optimal sequencing depth is a significant hurdle in the realm of whole genome sequencing. This depth is the backbone of obtaining high-quality genomic data, which directly influences the accuracy of variant detection and the overall reliability of genomic interpretation. However, several challenges arise as researchers endeavor to reach this ideal depth, compelling them to navigate through a maze of technical constraints and bioinformatics dilemmas.
Technical Limitations
The technical limitations associated with sequencing depth often stem from the intrinsic capabilities of current sequencing technologies. Sequencing machines have varying performance metrics, and none are without their limitations. Things like read length, error rates, and throughput play a crucial role in achieving sufficient depth.
For example, while Illumina platforms are widely favored for their high throughput, the short reads they produce can sometimes limit the ability to accurately assemble genomes, especially in complex regions. On the other hand, platforms like Pacific Biosciences may provide longer reads capable of spanning repetitive regions, yet their output might not match the sheer volume produced by other technologies. This trade-off necessitates a careful selection of the right technology, weighing depth against other variables such as cost and data quality.
"Balancing sequencing depth with the limitations of technology is akin to walking a tightrope - it requires precision and foresight."
Physical factors, such as sample quality and preparation techniques, can also impede the achievement of optimal depth. Samples that degrade during the preparation phase can lead to biases in depth, with certain regions being underrepresented. Moreover, library preparation protocols must be fine-tuned to ensure an even representation of genomic regions, making this step critical in avoiding pitfalls associated with low depth in certain areas.
Bioinformatics Challenges
Once the data is garnered from sequencing, the subsequent bioinformatics challenges can significantly impede efforts to glean meaningful insights from the genomic data. Data analysis pipelines must handle immense datasets, and with this comes the risk of not adequately accounting for the variability introduced by different sequencing depths.
Biologically relevant variants like Single Nucleotide Variants and Structural Variants demand precise detection and classification. Low sequencing depth can result in missed calls or false positives, complicating the interpretation for researchers. Effectively, without robust depth, it can become like searching for a needle in a haystack, making variant detection a daunting and often frustrating challenge.
The integration of varying datasets from multiple sources increases complexity as well. Different samples assessed under dissimilar parameters may lead to results that are not directly comparable. Addressing this variability requires sophisticated algorithms and extensive computational resources, which may not always be readily accessible, especially in less affluent research settings.
Additionally, bioinformatics tools must continuously evolve to keep pace with developments in sequencing technologies, necessitating ongoing education and resource investment for researchers. Maintaining a grasp on best practices in data processing and annotation becomes vital to avoid pitfalls that may skew results or lead to misinterpretations.
In summary, achieving optimal sequencing depth is beset with multifaceted challenges, from the limitations posed by sequencing technology to the intricacies of data analysis. Understanding and addressing these hurdles is essential for any study aiming for precision and reliability in genomic research.
Clinical Applications of Sequencing Depth
In the realm of genomic sciences, the clinical applications of sequencing depth hold significant importance. It's not merely about collecting extensive data but understanding how that data translates into tangible benefits for patient care and scientific advancements. The depth of sequencing impacts the reliability, sensitivity, and specificity of genomic analyses, which are pivotal in various clinical settings.
Personalized Medicine
Personalized medicine represents a paradigm shift in healthcare, where treatments are tailored to individual characteristics, especially genetic profiles. The depth of whole genome sequencing has a direct influence on identifying genetic variants that contribute to what makes each person unique.
For instance, in cases of rare genetic disorders, achieving an adequate sequencing depth allows for a clearer picture of patient genomes. Comprehensive coverage ensures that even low-frequency variants, which may have substantial clinical implications, are recognized. Patients can benefit from these insights through targeted therapies that align with their genetic makeup. Moreover, the selection of specific medications can be refined; for example, some drugs work better for individuals with specific genetic variants. This targeted approach minimizes adverse drug reactions and enhances treatment efficacy.
In practical terms, consider the case of pharmacogenomicsāunderstanding how an individualās genetic profile affects their response to medications. If sequencing depth is inadequate, thereās a risk of missing critical variants leading to unpredictable drug responses. Therefore, a depth thatās optimal for personalized medicine is not just a preference; itās essential.
Cancer Genomics
Moving onto cancer genomics, the implications of sequencing depth are profound. Cancer is often characterized by a multitude of genetic mutations that can vary significantly from one patient to another. Here, the quest for sufficient sequencing depth can uncover essential mutations that inform treatment decisions.
Detecting variants associated with tumor progression requires a careful balance between depth and breadth of the genomic data. For instance, some tumors harbor mutations only found at low frequency, making it crucial for sequencing depth to be high enough to identify these rare yet impactful genetic changes.
Furthermore, sequencing depth plays a pivotal role in assessing tumor heterogeneity, which refers to the genetic diversity within a tumor. Understanding this complexity can help in devising strategies for combined therapies that target multiple pathways, combating the tumor effectively.
"In cancer genomics, the accuracy of identifying mutations ultimately hinges on sequencing depth, guiding both diagnostics and treatment strategies."
Just as in personalized medicine, insights gained from cancer genomic studies can lead to significant advancements in targeted therapies. If a tumorās genetic landscape is thoroughly characterized through deep sequencing, clinicians can choose treatments that specifically target those mutations, enhancing patient outcomes.
In summary, the clinical applications of sequencing depth are pivotal in personalized medicine and cancer genomics. They elevate traditional treatment paradigms by integrating genomic data into patient care, fostering innovations that could lead to more tailored therapies and improved clinical outcomes. As our understanding of sequencing technologies and analytical tools evolves, the emphasis on achieving optimal sequencing depth will undoubtedly continue to increase.
Current Trends in Whole Genome Sequencing
As we peel back the layers of whole genome sequencing, a few current trends are clearly standing out. The increasing speed and accessibility of sequencing methods are not just buzzwords; they are reshaping how researchers and clinicians approach genomic studies. The dynamism of this field creates a fertile ground for new discoveries that can lead to tailored treatment plans and innovative research approaches, which is vital in todayās rapidly evolving scientific landscape.
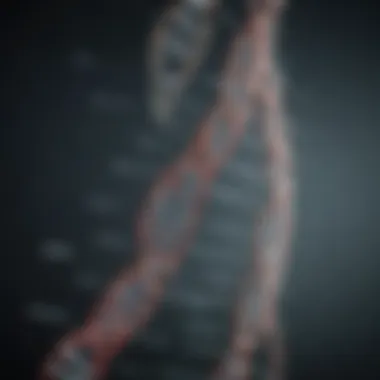
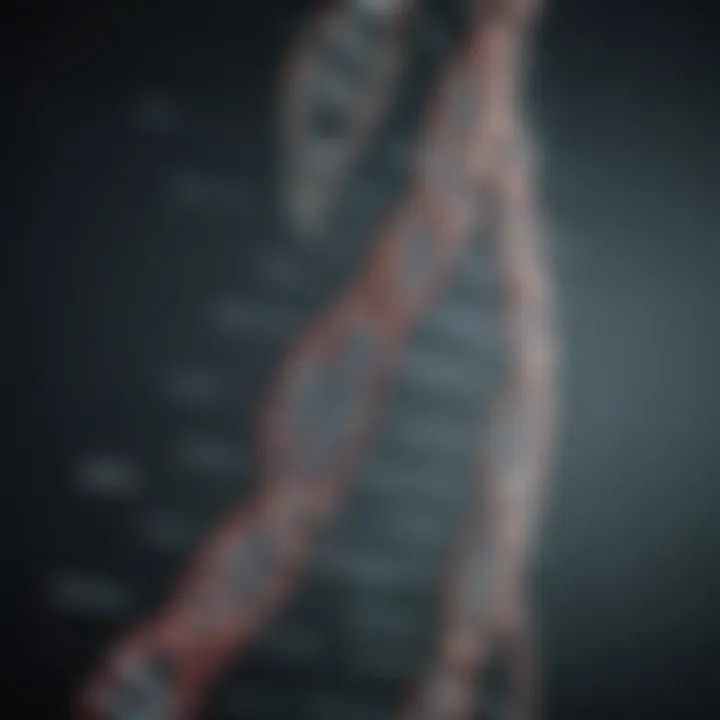
Next-Generation Sequencing Innovations
Innovations in next-generation sequencing (NGS) technologies have opened new doors for genomic research. Platforms are becoming faster, cheaper, and more accurate, enabling researchers to obtain comprehensive genetic information in a fraction of the time previously required.
For instance, Illumina's expanded sequencing capabilities now allow for higher throughput, which translates to gathering more data at once. Other platforms, like those from Pacific Biosciences, are paving the way for more precise long-read sequencing, which is essential when studying complex genomes or structural variations that are often missed with short-read technologies. The result? More reliable data that can drive important clinical decisions.
A few key trends include:
- Single-cell sequencing: This allows researchers to examine the genome of individual cells, improving our understanding of heterogeneity in tissues.
- Real-time sequencing: Technologies, such as Oxford Nanopore, permit immediate analysis of samples, facilitating rapid responses in clinical settings.
- CRISPR integration: Combining NGS with CRISPR gene editing provides a powerful tool for precise genetic modifications and understanding gene function.
"The revolution in sequencing technologies is not just transforming laboratories, but entire healthcare environments by paving the way for personalized medicine."
Data Integration Techniques
With the explosion of data generated by these sequencing innovations, effective integration techniques have become vital. The ability to synthesize vast amounts of genomic data with other types of biological and clinical data is critical for deriving meaningful insights.
Some emerging techniques in data integration include:
- Multi-omics approaches: These involve the simultaneous analysis of genomics, transcriptomics, proteomics, and metabolomics data. Combining these layers provides a more holistic view of biological processes.
- Artificial Intelligence and Machine Learning: Algorithms are being developed to handle large datasets, recognize patterns, and make predictions. This capability accelerates data analysis and enhances our understanding of genomic information.
- Cloud-based platforms: The shift towards cloud technologies allows researchers to store, share, and analyze genomic data collaboratively. This accessibility fosters a collective intelligence approach, where insights can be pooled from multiple research teams.
As these trends continue to evolve, they not only shape the direction of whole genome sequencing but also solidify its importance in diverse fields such as medicine and biology. Emerging technologies and integration techniques are rapidly making sequensing a central pillar in understanding complex biological systems.
Future Directions in Sequencing Depth Research
Advancing sequencing depth research is crucial for enhancing our understanding of genomics. As technologies evolve, the potential to glean more detailed and robust data from genomes is opening up new avenues for exploration in numerous fields. One of the most significant elements is how sequencing depth can impact both the quality of our findings and the efficiency of genomic analyses.
In light of recent developments, researchers are consistently seeking ways to leverage these advancements for improved genomic precision and insight. This ongoing evolution could lead to breakthroughs in various applications, whether in personalized medicine, hereditary disease understanding or even microbial diversity assessments. The implications of advancements in sequencing depth are profound.
Advancements in Sequencing Technologies
The strides in sequencing technologies are noteworthy. Take for instance the ongoing refinement of next-generation sequencing platforms. These systems are designed to produce more reliable sequence data at depth levels that were previously unattainable. By using innovative approaches like multiplexing, where multiple samples are sequenced simultaneously, scientists can increase throughput while effectively managing costs. This transition not only serves to enhance accessibility but also dramatically fosters a broader range of research initiatives.
Furthermore, technologies such as single-molecule sequencing could alter the landscape entirely. For example, both Pacific Biosciences and Oxford Nanopore Technologies focus on real-time sequencing and longer reads, which can lead to better detection of structural variants. Such capabilities allow for a comprehensive assessment of genomes, enabling researchers to harness a more complete picture.
- Key Technologies to Watch:
- Illumina Platforms
- Pacific Biosciences
- Oxford Nanopore Technologies
These advancements can bring long-term benefits not just in technical capacity, but also in enhancing the potential for discoveries in genomic research across diverse fields.
Ethical Considerations
While we marvel at the emerging advancements, it's crucial to ponder the ethical ramifications of these powerful tools. With the capability to analyze entire genomes at unprecedented depths, there arises a substantial responsibility to protect individuals' privacy and genetic information. As we'd all agree, no one wants their genetic data floating around without consent.
Moreover, the potential for misuse of genomic dataāthat is, events such as genetic discriminationādemands that we tread carefully. There's also the question of accessibility; not everyone can benefit equally from these technologies, leading to disparities in knowledge and healthcare. As such, itās essential for researchers and practitioners to engage with policy-makers and ethicists.
- Considerations for the Future:
- Data privacy and security measures
- Protocols for informed consent
- Addressing disparities in access to genomic technologies
Engaging with these ethical questions is fundamental in aligning our scientific advancements with societal values. Progress in sequencing depth research should not just be about technological capability but must also prioritize integrity and equality.
"The future of genomic research lies not only in the advancements we pursue but also in how we ethically navigate the realms they open up."
By thoughtfully addressing these aspects, the academic and scientific community can ensure that the future of sequencing depth research leads to not only groundbreaking discoveries, but also to responsible stewardship of our growing genomic knowledge.
Epilogue
In wrapping up, it becomes increasingly evident that whole genome sequencing depth is not just a technical detail, but a cornerstone of advancing our understanding of genetics. The implications of this topic stretch far beyond the laboratory bench, directly influencing fields like personalized medicine and evolutionary biology. As researchers, clinicians, and educators navigate the maze of genomic data, the significance of sequencing depth cannot be overstated.
A well-defined sequencing depth allows for accurate variant detection which can lead to significant breakthroughs in clinical diagnostics. For instance, when identifying rare genetic disorders, having sufficient depth is vital to ensure that all variants are accounted for, reducing the risk of overlooking critical mutations.
Summary of Key Insights
- Definition and Importance: This article has delineated what sequencing depth is, emphasizing its role in the overall quality and reliability of genomic studies.
- Technical Nuances: Various factors, including library preparations and sequencing technologies such as Illumina and Oxford Nanopore, significantly impact the sequencing depth outcomes.
- Applications Across Disciplines: We discussed the applications of sequencing depth in clinical genomics, population studies, and even microbial genomics. Each application underscores how critical depth is to the accuracy of results and subsequent insights.
- Future Prospects: Advancements in sequencing technologies signal a promising future where precision medicine can achieve better outcomes due to enhanced data quality, driven largely by optimized sequencing depth.
By laying out these insights, the article aims to equip students, researchers, and professionals with a better understanding of how to approach genomic challenges through an informed lens.
Final Thoughts
Looking ahead, the journey of whole genome sequencing depth seems bright yet fraught with challenges that need to be addressed. As we continue to push the boundaries of genomics, the deeper we delve into sequencing depth, the more the potential for discoveries unfurls.
"In genomic research, depth is not simply about quantity; it's about the quality of insights we can derive. Every base we sequence could unlock a world of biological secrets."
While the innovations on the horizon provide hope for resolving many current limitations, ethical considerations will play a crucial role in guiding how these advancements are applied in the real world. Understanding and navigating the implications of sequencing depth will ultimately dictate the success of future genomic studies.