Understanding Mass Spectrometry: A Detailed Guide
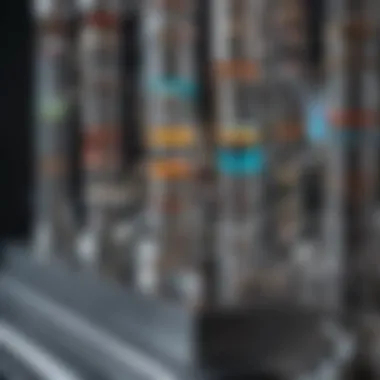
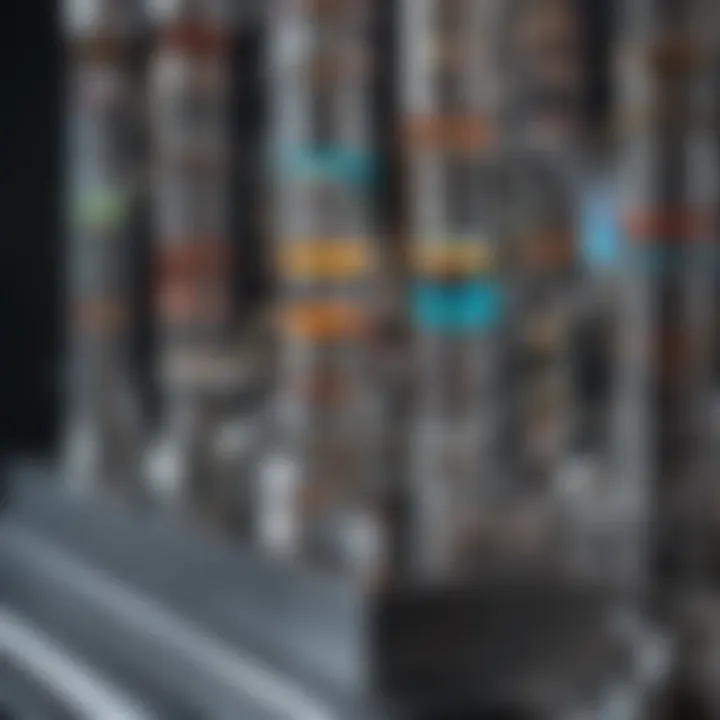
Intro
Mass spectrometry, a technique that has become indispensable in modern science, provides insights into the composition and structure of various compounds. Recognized for its precision and versatility, this analytical method is utilized across disciplines, including chemistry, biology, and environmental science. The aim of this article is to provide a detailed understanding of mass spectrometry, from its underlying principles to practical applications in research and industry.
In the coming sections, we will dissect the core principles of mass spectrometry and walk through the essential components of its instrumentation. Furthermore, we will highlight various data interpretation techniques and distinguish how this method can be applied in different scientific fields. By breaking down these concepts, we aim to enhance comprehension and encourage effective utilization of mass spectrometry in practical scenarios.
Article Overview
Exploring mass spectrometry involves grasping its foundational concepts as well as appreciating its wide applications. Begin by recognizing its significance in both academic and industrial settings, where it serves as a critical tool for compound analysis. From tracing metabolites in biological samples to detecting pollutants in environmental studies, mass spectrometry plays a nuanced role in understanding the world around us.
Summary of Key Findings
- Precision in Quantification: Mass spectrometry is known for its high-resolution ability to measure the mass-to-charge ratio of ions, allowing for the quantification of very low concentrations of substances.
- Versatile Applications: The methodâs flexibility accommodates a wide range of samples, from small organic molecules to large biomolecules, making it massively applicable in diverse research areas.
- Data Richness: The wealth of data generated by mass spectrometry can be interpreted in various ways, leading to deeper insights into compound structures and behaviors.
Research Objectives
This guide aims to:
- Clarify the principles and workings of mass spectrometry.
- Illustrate the instrumentation involved in conducting mass spectrometry analyses.
- Review the methods employed in data interpretation and analysis.
- Discuss real-world applications in multiple scientific fields.
Through this structured approach, we intend to demystify the complexities of mass spectrometry, serving both beginners eager to learn and seasoned practitioners looking to deepen their knowledge.
Prelims to Mass Spectrometry
Mass spectrometry stands as a foundational technique in analytical chemistry, acclaimed for its precision in measuring molecular weights and providing structural information about a wide range of compounds. Throughout the scientific community, this method has become indispensable in various applications such as drug development, environmental monitoring, and proteomics. Its ability to analyze complex mixtures with high sensitivity underscores its significance.
The importance of understanding mass spectrometry is two-fold. First, it equips researchers and practitioners with the skills to decipher intricate data that can illuminate pathways in chemical research and innovation. Second, grasping the underlying principles enhances the ability to critically evaluate experimental outcomes, which can be crucial in advancing scientific discourse. Analysts who master these concepts are better prepared to troubleshoot experimental issues, optimize methods, and interpret results effectively.
In this guide, we will explore the historical context and foundations of mass spectrometry to appreciate its evolution and its role in current scientific practices.
Historical Context
The roots of mass spectrometry trace back to the early decades of the 20th century. It was J.J. Thomson, the Nobel laureate, who first conceptualized the idea of utilizing electric and magnetic fields to separate ions by their mass-to-charge ratio. His resilience led to the construction of the first mass spectrometer, which paved the way for further advancements.
In the mid-20th century, the technique gained traction, especially in the field of organic chemistry. Notably, researchers began employing mass spectrometry to analyze natural products, leading to revelations about their structure and composition. The introduction of various ionization methods, like Electron Ionization (EI) and more contemporary ones such as Electrospray Ionization (ESI), enriched the capability of mass spectrometers considerably. Each new method brought an array of applications and refined the analysis strategies in numerous scientific domains.
Among its many contributions, mass spectrometry's role in identifying isotopes and quantifying organic compounds marked significant milestones, underlining its value in both academic and industrial laboratories.
Basic Principles
At its core, mass spectrometry operates on some fundamental principles that govern the movement and separation of ions. The process can be broken down into several stages, primarily: ionization, analyzation, and detection.
- Ionization: This initial step involves transforming neutral molecules into charged ions. Different techniques have been developed, with Electron Ionization being the classic mode, wherein high-energy electrons interact with gas-phase molecules. Alternatively, Electrospray Ionization allows for the ionization of larger biomolecules in solution, making it indispensable in biological analyses.
- Mass Analysis: Once ionized, the ions are accelerated through an electric field and directed into a mass analyzer. The mass analyzer separates ions based on their mass-to-charge ratio. For instance, the Time-of-Flight (TOF) analyzer measures the time it takes for ions to travel a fixed distanceâa method that reveals their mass.
- Detection: After separation, the ions are detected, generating a spectrum that displays relative abundance against mass-to-charge ratio (m/z). This spectrum provides critical insights into the molecular composition of the sample analyzed.
Understanding these principles allows scientists to not only interpret the resulting spectra but also manipulate variables during the process for tailored outcomes. The interplay of these stages renders mass spectrometry a truly versatile tool in modern analytical chemistryâan art and a science that demands both precision and creativity.
Instrumentation of Mass Spectrometry
The instrumentation of mass spectrometry serves as the backbone of this sophisticated analytical technique, impacting the quality and accuracy of the results obtained. Each component from ionization to detection plays a pivotal role in determining the success of a mass spectrometer's performance. Without a doubt, understanding these instruments and their functionalities is key for practitioners looking to fully leverage mass spectrometry's capabilities. This section delves into the diverse elements that make up mass spectrometry instrumentation, emphasizing the benefits and considerations that come with each.
Ionization Techniques
Ionization techniques are crucial because they transform the analyte into ions, allowing for further manipulation and analysis. The selection of a suitable ionization technique can greatly influence the efficiency and effectiveness of the overall mass spectrometry process. Below are some commonly used ionization methods.
Electron Ionization
Electron ionization (EI) stands out as one of the most established techniques in mass spectrometry, particularly within the realm of organic compounds. It utilizes high-energy electrons to knock off an electron from the analyte molecules, thus generating positive ions. One key characteristic of EI is its ability to produce a wealth of fragment ions, providing a comprehensive mass spectrum.
This technique is often favored due to its simplicity and robust performance when analyzing volatile compounds. However, electron ionization might not be ideal for larger biomolecules, as the fragmentation may obscure relevant spectral information. Despite this, its clarity in data presentation makes it a popular choice for many chemists.
Electrospray Ionization
Another significant technique is electrospray ionization (ESI). This method is especially notable for handling large biomolecules such as proteins and peptides. In ESI, the analyte is dissolved in a solvent and then sprayed at high voltage to create fine droplets, which rapidly evaporate and leave charged ions behind. A key characteristic of this method is its soft ionization nature, which helps in preserving the integrity of larger biomolecular structures.
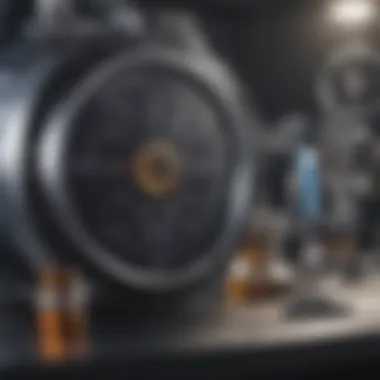
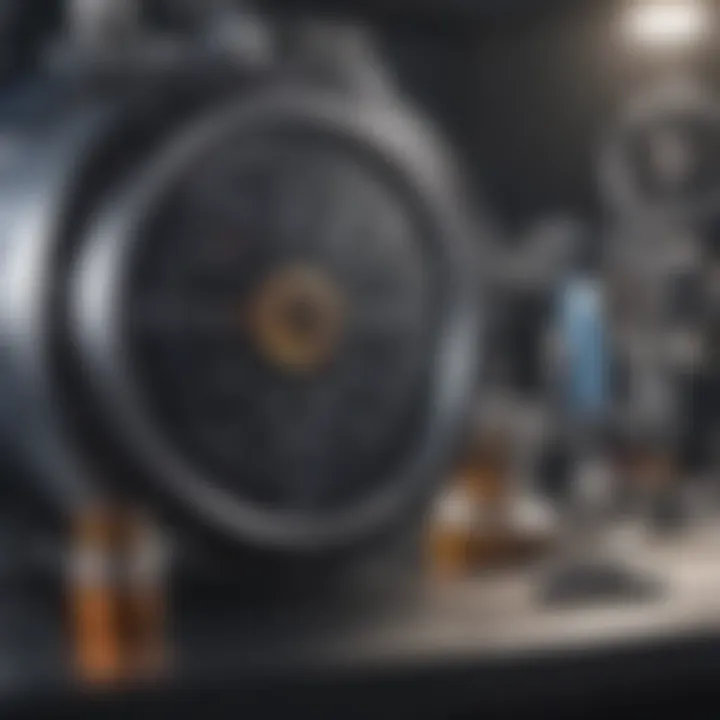
Electrospray ionization has garnered popularity largely due to its capability to analyze complex mixtures without prior separation. However, one disadvantage is that it may not be the most effective for small, non-polar molecules, which could limit its applicability.
Matrix-Assisted Laser Desorption/Ionization
The Matrix-Assisted Laser Desorption/Ionization (MALDI) technique offers unique advantages in mass spectrometry. It employs a laser to desorb and ionize molecules embedded in a matrix, usually a solid compound. This particular method allows for the analysis of large biomolecules and macromolecular complexes, which is crucial in fields like proteomics.
A standout feature of MALDI is its capability to handle samples of varying sizes and complex mixtures with relative ease. It produces clean spectra with minimal fragmentation, allowing for the observation of intact molecules, which is quite beneficial in profiling large compounds. However, significant upfront preparation and the potential for matrix interference can be obstacles in utilizing MALDI effectively.
Mass Analyzers
Once ions are formed, the subsequent step in mass spectrometry is analysis, handled by mass analyzers. These instruments separate ions based on their mass-to-charge ratio, and different analyzers provide varying levels of resolution and sensitivity. Understanding each type of analyzer enhances a practitioner's ability to select an appropriate tool for their specific research needs.
Quadrupole Mass Filter
The quadrupole mass filter is notable for its simplicity and versatility. It employs an oscillating electric field to allow only ions of a specific mass-to-charge ratio to pass through at any given time. The key characteristic that makes this analyzer appealing is its capability for rapid scanning of multiple ions, which provides efficient analysis of complex samples.
However, while quadrupole mass filters are reliable, their resolution is somewhat limited compared to other technologies, making them less suitable for applications requiring extremely high precision.
Time-of-Flight (TOF)
The Time-of-Flight (TOF) mass analyzer capitalizes on measuring the time it takes for ions to travel a known distance. The high speed of TOF analysis allows for high-resolution measurements, capturing detailed spectra quickly and accurately. Because of its ability to analyze a wide range of ion masses, it has become highly favored in contemporary mass spectrometry applications.
A unique feature of TOF is its capacity to accommodate large molecules with great detail, an attribute that's invaluable in proteomics research. Nonetheless, the initial setup and complexity could present challenges to some users.
Orbitrap
The Orbitrap mass analyzer is among the most advanced available, utilizing an electrostatic field to trap ions and measure their oscillation frequencies. One primary benefit of the Orbitrap is its high mass resolution and accuracy, making it suitable for identifying complex mixtures.
One of its key characteristics is its ability to generate very high-quality data at relatively low operational costs. However, the stability requirements and the need for advanced knowledge in operation can be barriers to entry for new users.
Detector Technologies
Finally, the detection of ions is the last critical element of mass spectrometry instrumentation. Accurate detection is essential for ensuring that the results obtained are reliable and reproducible. Two of the most commonly employed detection technologies in the realm of mass spectrometry are explored here.
Electron Multiplier
The electron multiplier is widely used due to its high sensitivity in detecting low-abundance ions. This technology amplifies the signal generated by incoming ions, enabling the detection of minute quantities of analytes, a key consideration in trace analysis.
A notable feature of electron multipliers is their rapid response time, making them ideal for time-sensitive analyses. However, they can exhibit limited dynamic range, which may restrict their efficacy in extremely high-concentration scenarios.
Ion Counting Detectors
Ion counting detectors, on the other hand, operate by measuring the number of ions directly, thus yielding precise quantification. Their robustness in quantifying ions across a dynamic range makes them highly beneficial for analytical applications requiring accuracy.
However, the trade-off typically comes in the form of lower sensitivity compared to electron multipliers, meaning that they may not be suitable when working with very low-concentration samples.
In summary, the instrumentation of mass spectrometry encompasses a variety of specific techniques and devices that together facilitate the analysis of an array of compounds. Each component, from ionization to detection, has its distinct advantages and drawbacks, impacting how scientists choose to apply mass spectrometry in their research. Understanding these elements not only aids in comprehension but also in the practical application of mass spectrometry in real-world scenarios.
Data Acquisition and Analysis
Data acquisition and analysis are paramount when it comes to mass spectrometry. This area not only involves collecting data from mass spectrometric instruments but also employing methods to analyze and interpret that data accurately. Effective data acquisition ensures that the mass spectra produced are representative of the sample being analyzed, while thorough analysis aids in deriving meaningful insights from the obtained results. This section explores the fundamental aspects of data processing and spectral interpretation, emphasizing their significance.
Basic Data Processing
Signal Processing
Signal processing is a critical aspect of mass spectrometry data analysis. Essentially, it involves the manipulation of raw data obtained from mass spectrometry to enhance the signals of interest while minimizing irrelevant noise. A key characteristic of signal processing is its potential to improve the clarity and quality of the data. This makes it a preferred choice for obtaining reliable results in mass spectrometry.
One unique feature of signal processing is its ability to apply algorithms that can filter out background noises and highlight true signals, allowing researchers to visualize their data in a more interpretive manner. However, one must tread carefully; over-processing can lead to the loss of valuable information. Thus, while it enhances data quality, it's crucial to maintain a balance to avoid misinterpretations.
Noise Reduction
Noise reduction focuses on eliminating or mitigating the unwanted electrical or signal noise that often accompanies mass spectrometry data. This factor is vital, as noise can obscure key information and lead to erroneous conclusions about the samples in question. A fundamental characteristic of noise reduction is its connection to enhancing data reliability and the overall accuracy of results. This makes it an indispensable technique in this article.
Noise reduction typically employs methods like averaging multiple scans or using advanced filtering techniques to suppress background interference. However, a drawback might be that excessive noise reduction could inadvertently alter the true nature of the signal, leading to potential misinterpretation of results. Striking a balance is necessary to safeguard data integrity while improving the reliability of analyses.
Spectral Interpretation
Mass Spectrum Analysis
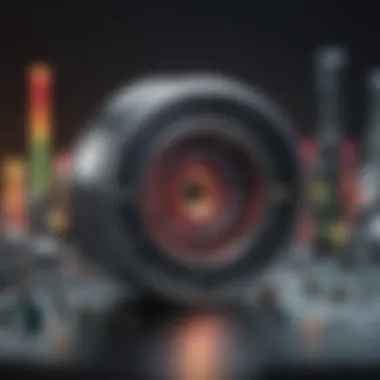
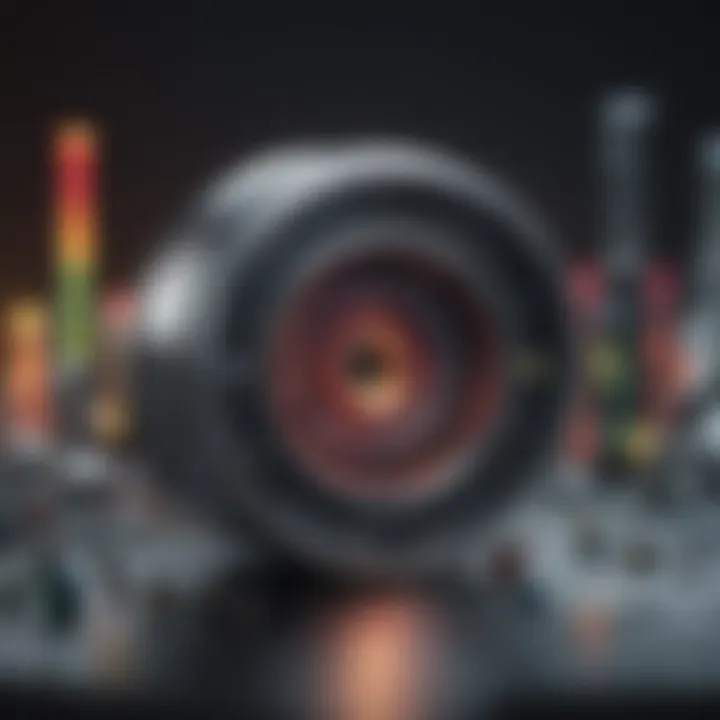
Mass spectrum analysis involves examining and interpreting the resultant mass spectrum generated by a mass spectrometer. This process contributes immensely to the identification and quantification of chemical compounds. A defining feature of mass spectrum analysis is its capability to provide a clear molecular fingerprint of the analyzed substances. This characteristic makes it a rewarding choice for those engaged in this field of study.
The unique aspect of mass spectrum analysis is its detailed depiction of mass-to-charge ratios (m/z) of ions, allowing researchers to deduce the composition and structure of unknown compounds. Nevertheless, interpretation necessitates a firm understanding of certain underlying principles; incorrect assumptions could lead to faulty conclusions and misguided research directions.
Identifying Peaks
Identifying peaks in a mass spectrum is essential for interpreting the data. Peaks correspond to ions detected in the sample and represent their abundance. This feature plays a critical role through which both qualitative and quantitative information can be gleaned from the mass spectrum. Identifying peaks is a significant focus within this article due to its direct impact on drawing conclusions from the data. The unique aspect of peak identification rests in its reliance on precise calculations and comparisons with known standards, helping distinguish between similar compounds. However, the challenge lies in the fact that overlapping peaks can complicate analyses. While peak identification serves as a linchpin for insightful findings, care must be taken to avoid misidentifying compounds, which could lead to serious misinterpretations in research.
Applications of Mass Spectrometry
Mass spectrometry (MS) serves as a cornerstone in various scientific domains. It enables researchers and professionals to analyze complex mixtures, identify unknown substances, and quantify targeted compounds. By taking advantage of the unique properties of molecules, mass spectrometry bridges gaps between fundamental science and practical applications. The versatility in its use across multiple fields demonstrates its significance within this discussion.
In Chemistry
Organic Compounds Analysis
Organic compounds analysis via mass spectrometry stands out due to its effectiveness in providing specific molecular weights and structure information. For instance, when chemists aim to identify complex organic molecules, MS shines in its ability to differentiate between isomersâsubstances with the same formula yet different structures, like glucose and fructose. This level of precision is paramount in organic synthesis and the development of new drugs. Its key characteristic lies in the clarity of mass spectra it produces, allowing for easy interpretation of data.
However, while mass spectrometry is an excellent tool for organic compound identification, practitioners must be mindful of the matrix effectsâinterferences from contaminants in the sampleâwhich can muddle results. This necessitates a careful selection of purification and concentration methods before analysis. Nonetheless, organic compounds analysis remains a widely-used approach due to its reliability in providing crucial insights within chemical research and applications.
Polymer Characterization
Turning our focus to polymer characterization, mass spectrometry plays a vital role in understanding the structure and composition of polymers. By analyzing the mass of polymer fragments, scientists can derive critical information about molecular weights and distribution, crucial for designing materials with desired properties.
The unique feature of polymer characterization lies in its ability to reveal molecular weight distribution, shedding light on the polymerâs behavior and performance in various applications, such as plastics and coatings. One might say itâs like putting together a jigsaw puzzle, where each piece reveals significant insights about the bigger picture.
Advantages include its precision and ability to analyze macromolecules, which some other methods struggle with. But, as with organic analysis, itâs important to remain cautious of limitations like ion suppression effects, which can lead to misinterpretation of results. Still, polymer characterization via mass spectrometry remains a go-to choice for chemists drawing insights from polymeric substances.
In Biology
Proteomics
When it comes to proteomics, mass spectrometry makes significant contributions by enabling the study and identification of proteins in complex biological systems. The profound characteristic here is how it can handle the diverse array of proteins present in cellsâeach with unique mass spectrometry signatures that can be analyzed.
Proteomics is beneficial not just for identifying proteins but also for analyzing post-translational modifications, which can impact protein function significantly. For example, phosphorylationâa common modificationâcan be easily detected through mass spectrometry, proving pivotal in signaling pathway studies and drug development.
Despite its advantages, challenges like variability in protein expression levels can complicate interpretation. Sensitive detection limits play a crucial role, as low-abundance proteins may not be easily spotted. Still, proteomics is essential in understanding life on a molecular level, making mass spectrometry a key player in biological research.
Metabolomics
Metabolomics, the study of small moleculesâmetabolitesâin biological systems, also benefits greatly from mass spectrometry. Using this technique allows researchers to capture a snapshot of biological activity at a specific time. This ability to analyze hundreds of metabolites in one go marks its significance.
A key characteristic of metabolomics is its application in disease marker discovery, allowing early-stage detection of conditions like cancer or metabolic disorders. Utilizing mass spectrometry, researchers can identify metabolic profiles associated with diseases, paving the way for personalized medicine approaches. Such breadth of information makes metabolomics a powerful companion to proteomics.
However, challenges do lurk in metabolomics. The complexity of biological matrices can interfere with analysis, and the reliance on accurate quantitation remains critical. This minor complication is not enough to overshadow the enormous potential of metabolomics, as it reveals critical insights into cellular processes and disease mechanisms.
In Environmental Science
Pollutant Detection
In the realm of environmental science, pollutant detection harnesses mass spectrometryâs capacity to identify trace levels of pollutants in water, air, and soil samples. Its crucial characteristic is its high sensitivity, enabling the detection of harmful substances at concentrations far lower than most traditional methods can manage.
The unique advantage of using mass spectrometry for pollutant detection lies in its quantitative analysis capability, allowing scientists to pinpoint not only what pollutants are present but also their concentrations. For instance, the detection of heavy metals in water sources can guide environmental policy and public health recommendations.
Yet, like any method, it's not without hurdles. Complex sample matrices can cause challenges in interpretation and quantification, meaning sample preparation must be performed with meticulous care. Nonetheless, the importance of pollutant detection using mass spectrometry cannot be overstatedâitâs a vital tool for preserving environmental and public health.
Biomonitoring
Biomonitoring reflects the use of mass spectrometry to track chemical exposures from various sources, assessing their effects on human health and ecosystems. This techniqueâs significance lies in its power to provide insightful data regarding biological responses to environmental chemicals.
A notable aspect of biomonitoring is its ability to analyze biological samplesâlike urine and bloodâensuring that even minimal exposure levels can be detected. By utilizing methods like urine metabolite profiling through mass spectrometry, researchers can draw correlations between environmental exposure and health outcomes.
However, challenges do arise in separating background levels from exposure-related signals, leading to potential misinterpretation of data. Despite this, biomonitoring continues to be a popular method within environmental research, offering crucial insights into the impact of environmental chemicals on health, guiding regulations and public policy decisions.
Mass spectrometry serves not just as a tool but as a bridge connecting various scientific realms, providing clarity amid complexity.
Challenges in Mass Spectrometry
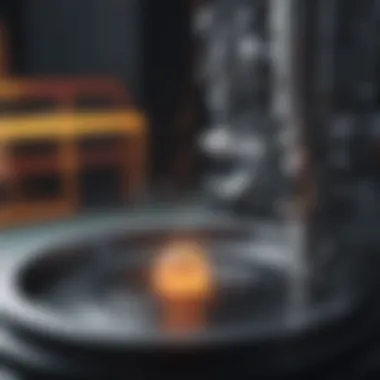
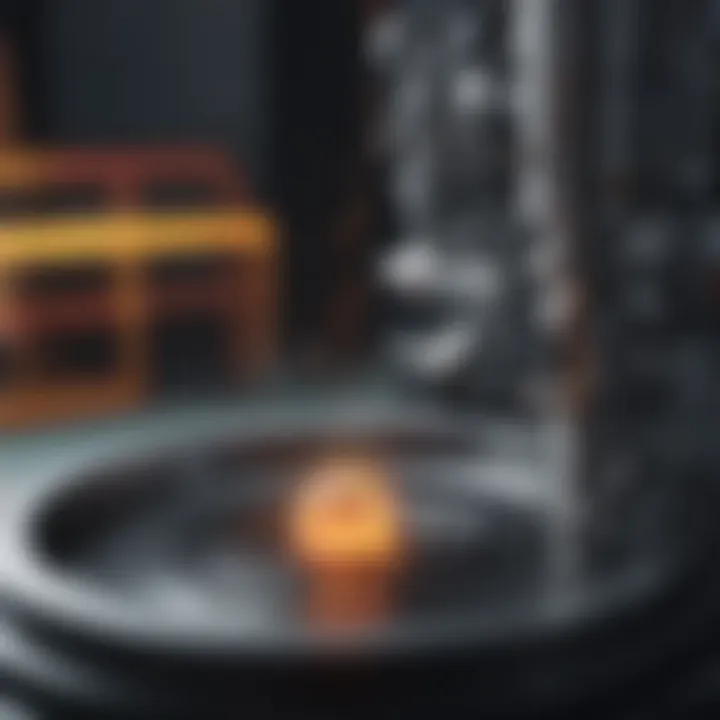
Understanding the challenges inherent in mass spectrometry is crucial for any practitioner in this field. This analytical method is a powerhouse in chemical analysis, yet it is not without its hurdles. Each challenge, rather than being merely an obstacle, can also serve as a catalyst for innovation and improvement in methodology. Addressing these challenges effectively can help refine analytical processes and improve the accuracy of results.
Let's dive into some of the significant challenges associated with mass spectrometry, focusing on matrix effects, sensitivity and detection limits, and the complications arising from complex sample matrices.
Matrix Effects
Matrix effects are one of the notorious challenges faced when interpreting mass spectrometry results. In this context, the 'matrix' refers to everything present in the sample aside from the target analytesâthat includes solvents, salts, proteins, and any other compounds. Essentially, these additional elements can interfere with the ionization process, ultimately skewing the measurement of target compounds.
- Ion Suppression or Enhancement: Sometimes, the presence of specific matrix components can inhibit the ionization of target compounds (ion suppression), resulting in lower signals than expected. Conversely, certain components may enhance the ionization, leading to inflated readings.
- Reproducibility Issues: Variability in sample composition can lead to inconsistent results, making it challenging to reproduce experiments in different labs or under different conditions.
- Mitigation Strategies: Strategies such as sample clean-up, the use of internal standards, and optimized calibration can help alleviate matrix effects. However, each method has its own limitations and may introduce new complexities.
Sensitivity and Detection Limits
Sensitivity and detection limits are paramount concerns in the realm of mass spectrometry. Essentially, sensitivity refers to the instrument's ability to detect low concentrations of an analyte, while detection limits indicate the smallest quantity that can be reliably quantified.
- Trade-offs: Increasing sensitivity often comes at a cost. For instance, optimizing an instrument for higher sensitivity may inadvertently affect its linear range, making it less reliable for quantifying higher concentrations.
- Analyte Characteristics: The structure of the target compound significantly influences sensitivity. For example, larger or less volatile compounds may yield lower signals, complicating detection efforts.
- Continuous Improvement: Ongoing advancements in technology aim to tackle these challenges. Innovations such as high-resolution tandem mass spectrometry are paving the way for improved detection limits and sensitivity.
Complex Sample Matrices
Analyzing samples with complex matrices presents a unique set of challenges that can often be tied back to both previous sections. Complex sample matrices can contain a multitude of interfering substances, making isolation of the target substance a considerable task.
- Sample Preparation: The need for meticulous sample preparation cannot be overstated. Techniques such as solid-phase extraction, liquid-liquid extraction, or even more advanced methods like microextraction, are often necessary to isolate analytes from undesirable components.
- Data Interpretation Difficulties: The intricate nature of these matrices can complicate data interpretation. Peaks in the spectra might not correspond to expected analytes due to interference or overlapping signals, presenting a challenging puzzle for researchers seeking clarity.
- Innovative Solutions: Employing multi-dimensional separation techniques can enhance specificity and resolution. These advancements ensure that professionals remain equipped to effectively analyze complex samples, improving both reliability and accuracy of results.
Ultimately, acknowledging and addressing these challenges not only enhances our understanding of mass spectrometry but also drives innovation in this field. As the analytical landscape evolves, overcoming these hurdles will be pivotal for advancing both research and practical applications.
Innovations in Mass Spectrometry
The field of mass spectrometry is not static; itâs a constantly evolving discipline that thrives on innovation. Ongoing advances significantly enhance the efficiency, accuracy, and breadth of this analytical technique. The thrust towards innovations in mass spectrometry is paramount, as it arms researchers with tools that can dissect chemical complexities at unprecedented levels. Whether itâs for routine laboratory analyses or groundbreaking research, advancements push the boundaries and open doors to applications that were once thought to be insurmountable.
Advances in Instrumentation
Instrumentation in mass spectrometry serves as the backbone for any analytical attempt. The recent surge in innovations here has birthed a range of cutting-edge devices that facilitate more accurate and efficient analyses. For instance, the introduction of high-resolution mass spectrometers provides scientists with the capability to distinguish between ions that differ by mere fractions of a dalton. This precision becomes essential in proteomics, where understanding minute distinctions between protein isoforms could reveal new biological insights.
Moreover, miniaturized mass spectrometers are gaining traction for their portability. The ability to carry a tool that once occupied an entire lab to the field can transform environmental monitoring. Just imagine, identifying pollutants right at the source with a handheld device! This evolving instrumentation plays a crucial role in multidisciplinary studies, linking environmental science with public health.
In addition, integrating tandem mass spectrometry (MS/MS) fundamentally redefines how we analyze complex mixtures. By providing enhanced fragmentation patterns, researchers can achieve deeper insights into molecular structures. The systematic approach unlocks pathways to designing new drugs, understanding metabolic pathways, or studying intricate molecular interactions.
Software Developments
The evolution of mass spectrometry doesnât solely hinge on hardware; software developments are equally influential. Analysis patterns in mass spectrometry have become increasingly data-driven, leveraging advanced algorithms and statistical tools. New software applications facilitate the handling of vast datasets generated by contemporary mass spectrometers, streamlining the interpretation process. These tools not only improve accuracy but also allow for quicker insights, making them indispensable in fast-paced research environments.
Developments in machine learning algorithms are particularly noteworthy. By analyzing trends in spectral data, these algorithms can predict outcomes or suggest probable matches to known compounds. The implications for drug discovery or forensic science, where identification of compounds in complex matrices can be cumbersome, are vast.
Moreover, graphical user interfaces have improved significantly. Researchers, regardless of their technical background, find themselves working more efficiently with intuitive platforms that present data visually. This ease of use allows for greater interdisciplinary collaboration, bridging the gap between chemists, biologists, and data scientists.
"Innovations in mass spectrometry not only refine existing methodologies but also expand the frontier of what questions we can ask about matter at its most fundamental level."
In summary, focusing on innovations in mass spectrometry reveals an exciting landscape marked by technological advancement. From sophisticated instruments to intuitive software applications, these innovations facilitate enhanced analytical capabilities across various domains. They empower the scientific community, driving research forward and enhancing our understanding of complex systems.
Future Trends in Mass Spectrometry
As the landscape of scientific research continues to evolve, mass spectrometry (MS) is on the brink of significant advancements. Understanding these future trends is crucial for any student, researcher, or educator in the analytical sciences. The relevance of these trends extends beyond mere novelty; they promise to enhance sensitivity, improve resolution, and increase throughput in various applications spanning chemistry, biology, and environmental science.
Emerging Techniques
The realm of mass spectrometry is witnessing a wave of emerging techniques that are poised to redefine its capabilities. One notable approach is desorption electrospray ionization (DESI), which allows for mass spectrometric analysis of solid samples directly. This is game-changing, particularly for the pharmaceutical field where surface analysis of drugs on various substrates can save time and resources.
Another fascinating technique is ambient mass spectrometry, which includes methods like Direct Analysis in Real Time (DART) or Desorption Electrospray Ionization. These allow rapid identification of compounds without extensive sample preparation. Not only does this speed up processes, but it also leads to accurate real-time results â essential in fields where every minute counts.
Moreover, single-cell mass spectrometry is emerging, enabling the analysis of individual cells rather than average populations. This precision offers profound insights, particularly in biology and medicine, where heterogeneity can dictate outcomes.
"The advancements in mass spectrometry techniques are not mere incremental changes but revolutionary shifts that can impact how researchers approach complex biological questions and environmental challenges."
Integration with Other Analytical Techniques
Integration with other analytical techniques is also shaping the future of mass spectrometry. As analytical problems become increasingly multifaceted, marrying mass spectrometry with other methodologies can yield richer datasets, ultimately enhancing the interpretive power of analyses.
Hyphenated methods such as GC-MS (gas chromatography-mass spectrometry) and LC-MS (liquid chromatography-mass spectrometry) are becoming industry standards. These approaches combine the strengths of separation techniques with the analytical finesse of mass spectrometry. This integration results in both qualitative and quantitative insights that are often unattainable through reliance on a single technique.
Furthermore, coupling mass spectrometry with NMR spectroscopy and infrared spectroscopy is gaining traction. By integrating these techniques, researchers can cross-validate findings, thus increasing the reliability of results. Such approaches ensure robust data collection which is critical in regulatory environments or in high-stakes research settings.
The merging of machine learning and artificial intelligence with mass spectrometry data analysis augurs a future where vast quantities of data can be processed with speed and accuracy. These technologies can identify patterns in complex datasets that a human analyst may overlook, thereby revealing insights that could climax in groundbreaking discoveries.