Mastering Oligo Annealing for Successful Cloning
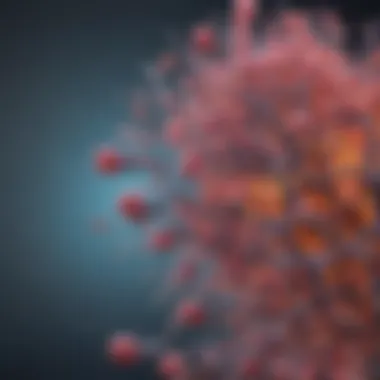
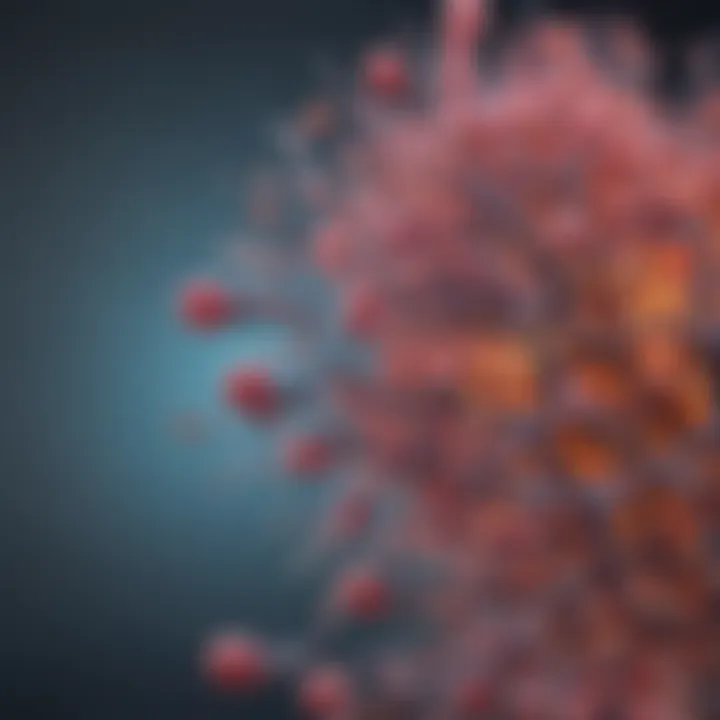
Intro
The process of annealing oligonucleotides is paramount in molecular cloning. Oligonucleotides, short strands of nucleic acid, play a crucial role in various stages of cloning workflows. They serve numerous functions, from priming DNA synthesis to facilitating hybridization reactions. This guide focuses on the process of annealing oligos, a vital step that ensures proper hybridization and enhances the effectiveness of subsequent cloning activities.
Understanding the principles of oligonucleotide design and thermodynamics is essential for researchers. This knowledge can facilitate the successful cloning of specific DNA sequences. A proper protocol not only streamlines the overall cloning process but also minimizes common mistakes that can lead to failed experiments. The aim is to equip scientists with insights that foster successful DNA manipulation.
With the right strategy, researchers can optimize their cloning efforts and increase the likelihood of obtaining accurate and reproducible results. Rigorous attention to detail during the annealing phase can significantly enhance the entire workflow, making this guide an important read for students, researchers, educators, and professionals in molecular biology.
Prelude to Oligonucleotides
Oligonucleotides, often referred to as 'oligos', play an essential role in modern molecular biology. Understanding what oligonucleotides are and their functionalities is crucial, particularly when discussing their application in cloning. In the context of this article, we aim to explore their design, influence, and implications on cloning processes.
Definition and Types of Oligos
Oligonucleotides are short sequences of nucleotides, typically composed of 13 to 25 bases. These sequences are synthesized to match specific regions of DNA or RNA. Their flexibility in design allows for the accommodation of various experimental needs, making them a fundamental tool in molecular biology.
There are several type of oligonucleotides:
- DNA Oligonucleotides: These are the most common and are utilized for PCR amplification, sequencing, and hybridization.
- RNA Oligonucleotides: Often used in antisense therapies and ribozyme studies.
- Modified Oligonucleotides: These come with chemical modifications to enhance properties like stability and binding affinity.
By selecting the appropriate type of oligonucleotide, researchers can tailor their experiments to meet specific objectives, yielding better results.
Role in Molecular Cloning
In molecular cloning, oligonucleotides serve as the foundation for introducing foreign DNA into host cells. They act not only as primers in polymerase chain reactions (PCR) but also function as adaptors that allow the ligation of DNA fragments. Properly designed oligonucleotides can significantly increase cloning efficiency and specificity.
Additionally, oligonucleotides help facilitate the amplification of target regions, which can then be used in various downstream processes such as sequencing and expression analysis. The versatility of oligonucleotides extends their role beyond just molecular cloning, enhancing tools such as gene editing and diagnostics. Thus, understanding oligos is not only important for cloning but also vital for many contemporary biotechnological applications.
"The precision of oligonucleotide design can determine the success of an entire molecular biology experiment."
As we continue, we will further explore the basic principles of annealing and how these principles directly relate to the design and application of oligonucleotides in cloning.
Basic Principles of Annealing
Understanding the basic principles of annealing is paramount for researchers involved in molecular cloning. This section elucidates the crucial aspects that govern the annealing process. By appreciating these principles, scientists can optimize their cloning workflows, mitigate errors, and enhance the fidelity of their experiments. Proper annealing techniques ensure that oligonucleotides bind effectively to their complementary strands—an essential step for successful ligation.
Mechanism of Annealing
The annealing process involves the binding of two complementary strands of nucleic acids. This occurs through base pairing, where adenine pairs with thymine, and cytosine pairs with guanine. The stability of the resulting double helix is influenced by the base composition and length of the oligonucleotides.
During cooling from high temperatures, the oligos collide more frequently. When complementary strands meet, they can hybridize if conditions are favorable. The key here lies in the temperature. A high temperature may separate the strands, while a lower temperature allows for hybridization. This critical transition defines the annealing phase. The rate of this process can be affected by several factors, including initial oligo concentration and the presence of salts, which stabilize the DNA structure.
Good practices during this phase include a gradual temperature drop to avoid non-specific binding. This controlled cooling not only enhances specificity but also minimizes the formation of secondary structures that can interfere with cloning.
Factors Influencing Annealing
Multiple factors can influence the annealing efficiency and specificity of oligonucleotides. Some of these are:
- Oligo Concentration: Higher concentrations can lead to increased hybridization events but may also elevate the risk of non-specific binding. Finding a balance is crucial.
- Temperature: Annealing temperatures should be optimized. Variations in temperature can significantly impact the efficiency of the annealing process. A good starting point is around 5 degrees Celsius below the melting temperature (Tm) of the oligonucleotides.
- Salt Concentration: Ionic strength can assist in stabilizing the double helix. Too much or too little salt can disrupt annealing.
- Sequence Length and Complexity: Longer oligos require more precise conditions for successful annealing. Moreover, sequences with repetitive patterns might form secondary structures that can hinder effective binding.
Proper consideration of these factors will not only enhance the cloning success rate but also streamline the entire experimental design.
In summary, understanding and applying the basic principles of annealing is foundational for anyone working with oligonucleotides in cloning. By mastering these mechanisms and recognizing the factors at play, researchers can significantly improve their cloning outcomes.
Designing Oligonucleotides for Cloning
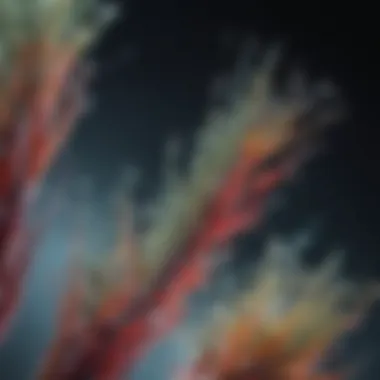
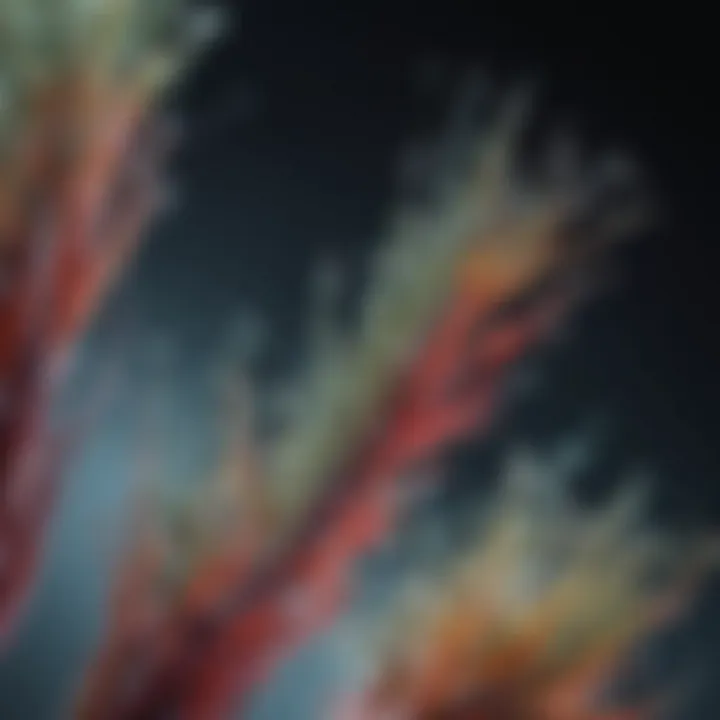
Designing effective oligonucleotides for cloning purposes is vital for successful experiments in molecular biology. The characteristics of each oligonucleotide can significantly affect the efficiency of the cloning process, influencing both yield and specificity of the desired products. It is essential to carefully consider various aspects during the design phase to ensure optimal results.
Length and Composition
The length and composition of an oligonucleotide are primary determinants of its hybridization efficiency and specificity. Typically, oligonucleotides for cloning range between 18 and 30 bases in length. Shorter oligonucleotides may not bind as stably, while excessively long ones increase the risk of mishybridization. Moreover, the nucleotide composition plays a crucial role. A balanced ratio of adenine, cytosine, guanine, and thymine contributes to favorable melting temperatures, which in turn impacts the annealing efficiency.
Additionally, incorporating G or C rich segments can enhance thermal stability. These bases pair stronger than A or T bases and increase the overall performance of the oligonucleotide during cloning. Therefore, it is wise to focus on both length and nucleotide distribution when crafting oligonucleotides.
Inclusion of Restriction Sites
The inclusion of restriction sites within oligonucleotide design is an important consideration for cloning applications. Restriction enzymes are essential tools that allow for precise cutting of DNA at specific sequences. By incorporating these sites into the oligonucleotides, researchers ensure that their target sequences can be efficiently cloned into vectors. This improves the flexibility in creating recombinant DNA.
However, careful selection of restriction sites is necessary. It is crucial to avoid internal restriction sites within the region of interest to prevent unwanted cleavage. Additionally, the chosen enzyme should have compatible overhangs that facilitate ligation into the plasmid or vector of choice.
Avoiding Secondary Structures
Secondary structures in oligonucleotides can hinder annealing and the overall effectiveness of cloning. Hairpin loops, self-dimers, or other complex formations can occur due to complementary sequences within the oligo. These structures may prevent the oligonucleotide from hybridizing with the target sequence, negatively affecting the success of the cloning process.
To mitigate this risk, various software tools can analyze potential secondary structures by simulating folding patterns. Researchers should select oligonucleotide sequences that minimize these interactions. It may also be beneficial to utilize modified nucleotides if needed, as they possess properties that may influence the formation of secondary structures.
By addressing length, composition, restriction sites, and secondary structures early in the design process, researchers are more likely to achieve successful cloning outcomes.
Thermodynamics of Oligo Annealing
Understanding the thermodynamics involved in oligo annealing is crucial for successful cloning experiments. The thermal properties of oligonucleotides significantly influence their ability to hybridize properly. Several factors, including the stability of the DNA duplex formed, dictate the overall success of the cloning procedure. Having a strong grasp of these thermodynamic principles leads to optimized conditions, which in turn enhances reproducibility and reliability in molecular cloning applications.
One central aspect of this topic is the melting temperature (Tm), which helps assess how robust the oligonucleotide interaction will be under varying conditions. Knowing how to calculate and interpret Tm is essential for determining appropriate annealing temperatures, which ensure efficient hybridization without mispairing or nonspecific interactions.
The thermodynamics of oligo annealing encompasses several significant benefits. By understanding these concepts, scientists and researchers can:
- Achieve higher fidelity in the cloning process,
- Minimize the risk of nonspecific binding,
- Tailor protocols to different experimental needs, and
- Enhance overall success rates in cloning experiments.
Understanding Melting Temperature (Tm)
Melting temperature (Tm) is defined as the temperature at which half of the oligonucleotide strands are in the double-helix form and half are in the "melted" single-strand form. This metric serves as a critical reference point when setting annealing temperatures for oligos.
Tm can be influenced by various factors, including:
- Oligo Length: Generally, longer oligonucleotides have higher Tm values due to increased base stacking interactions.
- GC Content: Oligos rich in guanine and cytosine will have higher Tm values, as these pairs form three hydrogen bonds, compared to the two hydrogen bonds formed by adenine and thymine pairs.
- Salt Concentration: Higher salt concentrations stabilize DNA duplexes, thereby increasing Tm.
It is important to use Tm values in calculations because they provide insight into the temperature regimen needed during the annealing phase. While different formulas exist for calculating Tm, a common one is:
Key Considerations for Tm
- Ensure that Tm calculations are accurate for the oligos involved.
- Keep in mind how Tm varies with experimental setups and conditions. Adjustments to Tm may be necessary based on buffer composition.
Calculating Optimal Annealing Temperature
Calculating the optimal annealing temperature (Ta) helps ensure effective hybridization of the target sequences. The Ta should ideally be set a few degrees below the Tm of the oligo to facilitate specific binding during the amplification process. This temperature ensures that the interaction remains stable without promoting mismatched binding.
For effective calculations, a common practice is:
- Calculate the Tm for both forward and reverse primers.
- Take the average of these Tm values.
- Subtract 3 to 5 °C from this average to determine the optimal Ta.
When developing protocols, always consider the specificity of the oligos being used and adjust the annealing temperature accordingly. Properly optimizing Ta improves the overall robustness of the cloning experiment and can lead to significant enhancements in results.
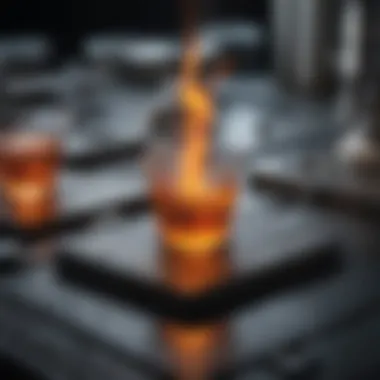
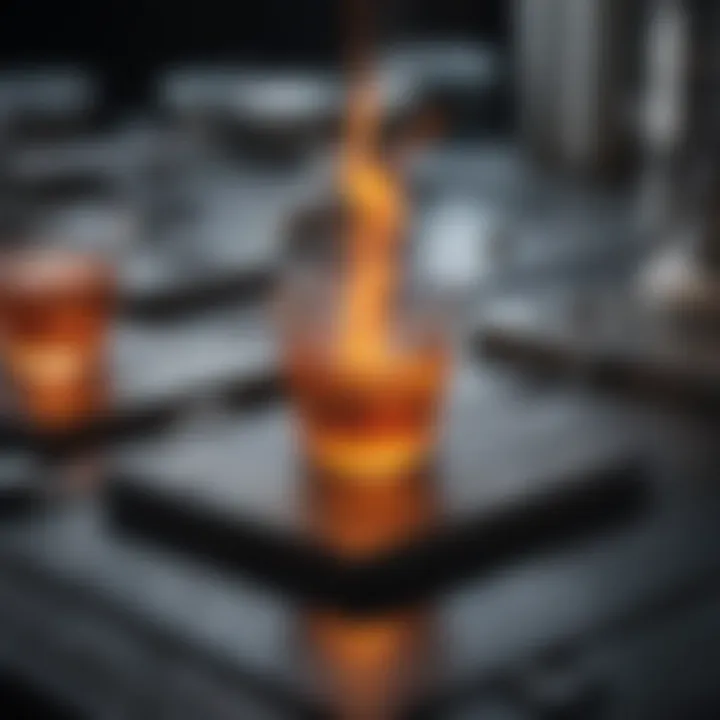
In sum, thorough comprehension of the thermodynamics governing oligo annealing is instrumental in achieving cloning success. This knowledge allows for precise manipulations of experimental conditions, leading to reliable and effective molecular cloning outcomes.
Protocols for Oligo Annealing
The use of oligonucleotides in molecular cloning is fundamental. Protocols for oligo annealing directly impact the success rates of cloning experiments. By following well-established protocols, researchers can minimize errors and creating highly reliable DNA constructs. Proper annealing conditions ensure that the oligonucleotides hybridize correctly, facilitating subsequent ligation and amplification steps. This section will discuss standard annealing protocols and temperature cycling methods, both of which are essential in achieving optimal conditions for oligo hybridization.
Standard Annealing Protocols
Standard annealing protocols typically involve a few key steps that are crucial for ensuring effective oligo hybridization. First, a mixture of complementary oligonucleotides must be made, which are usually designed based on the target sequence. The concentration of these oligos often plays a key role in achieving optimal results. Higher concentrations can lead to increased hybridization rates, but if too concentrated, it could result in non-specific binding or secondary structures, which negatively affect ligation success.
Once the oligonucleotides are mixed, the temperature must be accurately controlled. The mixture is slowly heated to a temperature that is typically slightly above the calculated melting temperature (Tm) of the oligos, fostering denaturation. Following this, the solution temperature is slowly decreased to allow for annealing. A slow cooling process helps to promote the formation of stable duplexes.
It is also important to maintain a conducive buffer environment, often with Tris or another stabilizing agent, to prevent degradation of the oligos. The choice of buffer should reflect the specific requirements of the downstream applications.
Temperature Cycling Methods
Temperature cycling methods are another effective approach for oligo annealing. This method involves multiple rounds of heating and cooling in a controlled manner. It allows researchers to enhance the specificity and efficiency of the annealing process.
A typical temperature cycling protocol may start with heating the reaction to a high temperature to denature any existing structures. This is followed by rapid cooling to a lower temperature for annealing, and then a moderate temperature step to stabilize the formed duplexes.
The advantage of temperature cycling is its adaptability. Researchers can optimize individual cycles based on the specific oligonucleotide sequences involved. The cycling method can help to selectively enrich only those hybridized oligos, reducing the chance for poor quality products.
Proper protocol adherence is key to enhancing the probability of successful cloning outcomes.
In summary, employing standard annealing protocols or temperature cycling methods can greatly impact the efficiency of oligator hybridization. The selection of an appropriate protocol should be dictated by the specific design and purpose of the cloning project. Understanding and implementing these techniques is fundamental for any researcher engaging in oligo annealing for cloning applications.
Common Pitfalls in Oligo Annealing
Annealing oligonucleotides is a critical step in nucleic acid research and cloning. However, there are common pitfalls that can severely affect the outcome of the cloning process. Identifying these pitfalls allows researchers to troubleshoot and refine their approaches, ensuring more reliable results. Here, we dive into two significant issues that often arise during oligo annealing: over-annealing and improper oligo concentration. Understanding these pitfalls helps to enhance overall experimental success.
Over-annealing Issues
Over-annealing occurs when oligonucleotides bind too tightly or at an incorrect temperature during the annealing process. This can lead to stable single-stranded regions that prevent proper hybridization with the target DNA. The implications of this issue can be extensive. When oligos fail to hybridize effectively, it can result in low yield and purity of the desired product.
Some factors contributing to over-annealing include:
- Excessive annealing time: Prolonging the annealing period can lead to increased binding stability, creating issues with specificity.
- Higher than necessary temperatures: Using temperatures above the optimal range can inadvertently promote unintended interactions between oligos.
To mitigate these problems, researchers should closely monitor both the temperature and duration of the annealing process. Employing a protocol that includes a preliminary temperature ramp can help achieve a more controlled and precise annealing reaction.
Improper Oligo Concentration
Another common issue is improper oligo concentration. This can be a double-edged sword, as both overly high and excessively low concentrations can adversely affect hybridization efficiency. Not finding the right balance can lead to suboptimal results in cloning experiments.
Signs of improper oligo concentration include:
- High concentrations: Can cause non-specific binding, increasing background noise in assays. As a consequence, researchers may encounter false positives or misleading results.
- Low concentrations: May lead to inadequate hybridization, resulting in poor amplification and low yields of the target DNA.
To address this issue, a well-established practice is to perform a titration experiment. This method evaluates different concentrations of oligonucleotides to determine the optimal amount for a given application. Such a systematic approach contributes to better efficiency in cloning and ultimately enhances the chances of obtaining a successful outcome.
Regularly reviewing your annealing protocols while paying attention to these common pitfalls will improve your cloning efficiency and reliability.
Understanding these pitfalls empowers researchers to refine their oligo annealing strategies, fostering improved outcomes in their molecular biology projects.
Enhancing Cloning Success Rates
Enhancing cloning success rates involves understanding how specific strategies and conditions in oligonucleotide handling impact the overall efficiency of cloning experiments. This section underscores the importance of precise control over oligonucleotide quality and ligation conditions to achieve better outcomes in molecular cloning. Ultimately, improved success rates in cloning can lead to more reliable research findings and facilitate progress in various fields including genetics, therapeutic development, and synthetic biology.
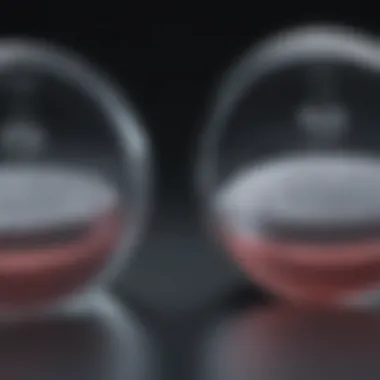
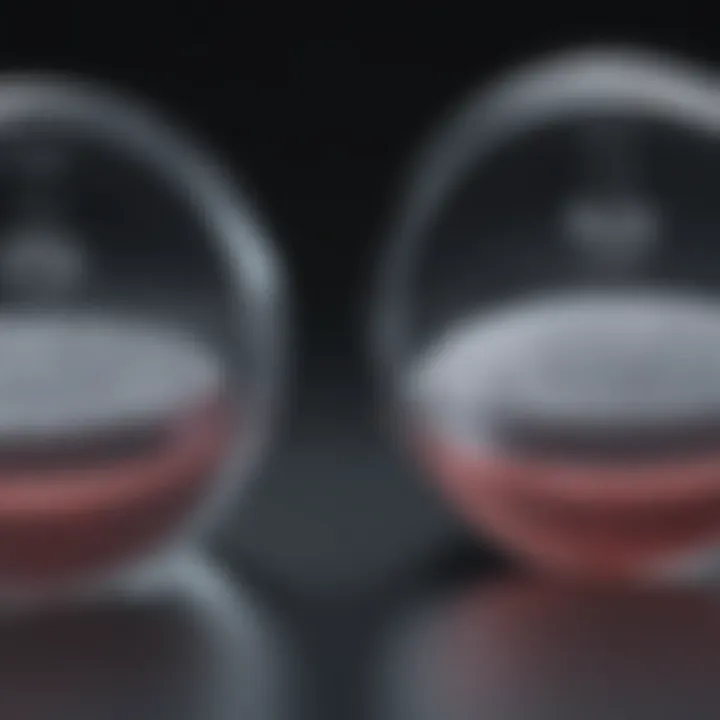
Quality Control of Oligonucleotides
Quality control of oligonucleotides is a critical aspect in the context of cloning. Poorly synthesized oligonucleotides can lead to numerous issues, such as inefficiency in hybridization and unexpected results during ligation. Ensuring high quality involves several factors:
- Purity: Oligonucleotides must be devoid of contaminants and truncated sequences. Higher purity leads to better performance in cloning.
- Length Consistency: Variability in oligonucleotide length can affect hybridization behavior. It is crucial to maintain consistency within stipulated length ranges.
- Functional Integrity: The presence of functional groups, such as phosphorothioates or modifications for stability, should also be verified.It is advised to utilize analytical techniques, such as HPLC (High Performance Liquid Chromatography) or mass spectrometry, for verifying oligonucleotide quality. These techniques can identify any impurities or variations in sequence, which may otherwise lead to inefficient cloning. Additionally, employing commercial sources that guarantee oligonucleotide quality can mitigate risks associated with home-synthesis.
Optimizing Ligation Conditions
Optimizing ligation conditions is paramount to enhance the efficiency of cloning experiments. The ligation reaction's success greatly depends on factors like enzyme concentration, temperature, and incubation time. Here are key considerations:
- Enzyme Selection: Choosing the right ligase, such as T4 DNA ligase, is critical. Each ligase has different properties that can influence the efficiency of ligation.
- Molar Ratios: Ensuring appropriate molar ratios between the vector and insert is necessary. A commonly suggested ratio is three times the molar concentration of the insert compared to the vector.
- Incubation Temperature: The temperature for the ligation reaction should be optimized. Generally, room temperature reactions are suitable, but some protocols suggest incubating at 16°C overnight, as it allows for more efficient binding.
- Time Consideration: The duration of the ligation reaction can vary. Some researchers may find success with 30 minutes to a few hours, while others opt for overnight ligation.
Utilizing these optimized conditions can greatly improve the likelihood of successful ligation and transformation into host cells.
"Quality control and optimized ligation conditions are indispensable for successful cloning. Without them, even well-designed oligonucleotides may yield poor results."
In summary, enhancing cloning success rates is largely a function of meticulous quality control and thoughtful optimization of ligation conditions. By integrating these strategies into cloning practices, researchers can attain higher success rates, thereby fostering reliable outcomes in their experimental endeavors.
Case Studies and Applications
Understanding the practical applications of annealing oligonucleotides is essential for researchers and practitioners in the field. This section sheds light on real-world scenarios where oligo annealing has led to successful cloning, as well as examines failures that provide crucial lessons.
Successful Cloning Examples
Cloning experiments are often validated through case studies that demonstrate effective annealing techniques. For instance, a prominent example is the cloning of green fluorescent protein (GFP) into a plasmid vector. Researchers designed specific oligonucleotides that included complementary sequences to the target gene and appropriate restriction sites. The careful selection of temperature during the annealing phase was critical, allowing the oligos to hybridize consistently.
The success of this experiment can be attributed to several factors:
- Optimal oligo design: The use of high-quality, well-characterized oligonucleotides minimizes variability.
- Meticulous adherence to protocols: Following established temperature cycling methods ensures reliable results.
- Thorough pre-cloning analysis: Running simulations to confirm Tm and reaction efficiencies.
Each successful cloning instance adds to the pool of knowledge, revealing the importance of rigor in oligo synthesis and experimental execution. Such cases are essential for demonstrating the viability of innovative cloning strategies.
Lessons Learned from Failed Experiments
Failures are rich in educational value and can guide future efforts in cloning. One notable case involved attempts to clone a large gene fragment where the oligonucleotide design was not optimized. The oligos formed secondary structures that impeded effective annealing. Numerous trials yielded no results, triggering a systematic review of the methodologies used.
Some critical takeaways from failed cloning experiments include:
- Importance of secondary structure evaluation: Avoiding structures that could interfere with hybridization is vital. Tools like Mfold can assist in visualization.
- Concentration matters: The oligos used were at low concentrations, which can lead to incomplete annealing and inefficient ligation.
- Iterative testing: Adjusting parameters based on past failures often leads to breakthroughs. Small changes to temperature or oligo concentrations can lead to improved outcomes.
Future Directions in Oligonucleotide Technologies
The field of oligonucleotide technologies continuously evolves, reflecting the growing complexity and demands of molecular biology. Understanding these future directions is crucial for researchers aiming to enhance their cloning strategies and streamline experimental processes. Key considerations include advancements in design tools and innovations in cloning techniques, each contributing to improved outcomes in genetic engineering.
Emerging Trends in Oligo Design Tools
Innovations in oligo design tools play a pivotal role in optimizing oligonucleotide creation. With the rise of computational biology, sophisticated software solutions have become indispensable. These tools now offer features such as:
- Automated analysis of potential secondary structures, reducing complications during hybridization.
- Customizable parameters for Tm calculations, ensuring optimal conditions for experiment-specific needs.
- Comprehensive databases of existing sequences that facilitate better design efforts by allowing researchers to compare and contrast their designs against established standards.
Moreover, recent trends indicate a move toward integration with artificial intelligence, enhancing predictive capabilities for oligo efficiency. Utilizing machine learning algorithms, these tools can offer insights into the biological behavior of designed oligos based on historical data, significantly improving the success rates of cloning experiments. As such resources become more prevalent, researchers must align their methodologies with these emerging technologies to maximize their cloning potential.
Innovations in Cloning Techniques
Cloning techniques have also seen substantial innovations, fortified by advancements in oligonucleotide applications. Noteworthy innovations include:
- CRISPR technology, which has escalated the speed and precision of genetic modifications. Oligonucleotides can be tailored as guide RNAs to ensure targeted edits are effective and efficient.
- Next-generation sequencing (NGS) facilitates a deeper understanding of oligo performance, enabling better assessments of cloning fidelity.
- In vitro transcription and translation systems have developed, allowing for the rapid generation of proteins from cloned genes, bridging oligonucleotide technologies and protein research.
These techniques not only improve efficiency but also expand the scope of what is possible in cloning. Understanding these advancements will enable researchers to adopt practices that leverage the best available technologies to enhance the reliability of their experiments.
"Integration of next-generation sequencing with traditional cloning methods can help in validating the outcomes more effectively, thus reducing the potential for errors."
To summarize, the future directions in oligonucleotide technologies present significant opportunities for enhanced cloning practices. By keeping abreast of these innovations, researchers can effectively tailor their approaches, driving forward the boundary of what is achievable in molecular biology.