The Evolution and Impact of Semiconductor Technology
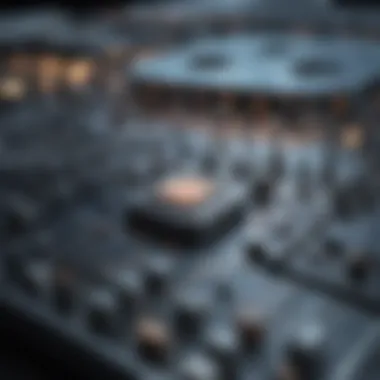
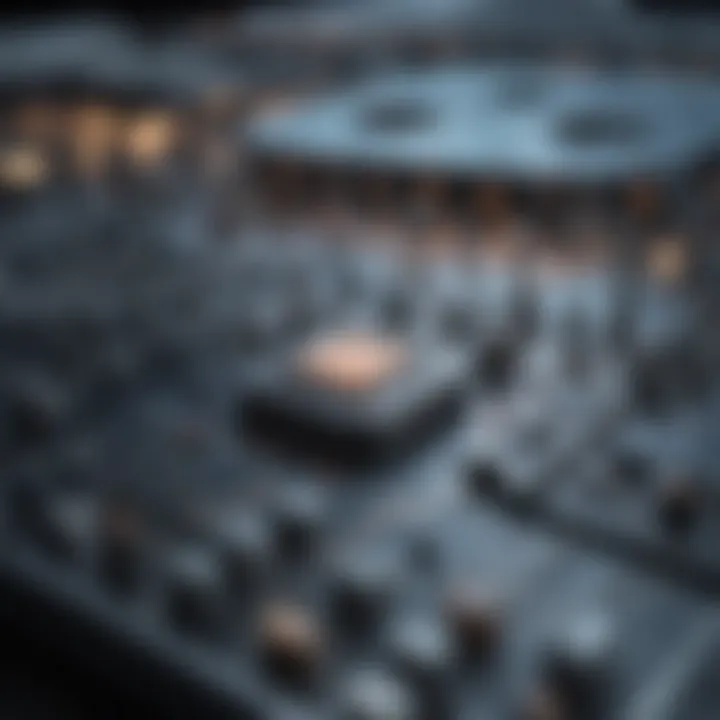
Intro
Semiconductor technology stands as the backbone of modern electronics and computing. From smartphones that fit in our pockets to supercomputers powering vast data centers, this technology catches the unseen currents that drive the digital age. But what exactly is a semiconductor? In layman's terms, semiconductors are materials that can both conduct and insulate electricity, depending on the circumstances. This unique property allows them to serve a multitude of functions in electronic circuits.
As we venture deeper, it becomes evident that the evolution of semiconductor technology has been nothing short of revolutionary. Starting from the early days of vacuum tubes, we have transitioned to transistors, and now we find ourselves amidst the rise of nanotechnology and quantum computing. The shift towards smaller, faster, and more efficient devices has reshaped not only the marketplace but also our day-to-day lives.
In this article, we will trace the journey of semiconductor technology from its modest beginnings to its pivotal role today. We'll delve into its core materials, the complex processes of fabrication, and the hurdles it faces in the modern era. Let's embark on this illuminating exploration, understanding that each silicon chip plays a critical role in shaping our digital experiences.
Article Overview
Summary of Key Findings
The core exploration of this article revolves around three key themes:
- The historical progression of semiconductor technology and its transformative impact on computing.
- Technical advancements and the future trajectory of semiconductors, especially concerning nanotechnology.
- The chief challenges facing the industry today, including supply chain issues and sustainability concerns.
These elements together provide a comprehensive perspective, offering insights into how semiconductor technology continues to evolve.
Research Objectives
This article aims to:
- Illuminate the history and foundational principles of semiconductor technology.
- Analyze recent advancements and the implications of emerging technologies like nanotechnology, which has shifted paradigms in the industry.
- Discuss key industry challenges and evaluate responses from major players and emerging trends impacting the semiconductor landscape.
By achieving these objectives, we hope to shine a light on the significance of semiconductors not just in technology but in the everyday lives of people around the world.
Foreword to Semiconductor Technology
Semiconductor technology stands at the core of the modern electronic age. Nearly every device we rely on—from mobile phones to advanced computing systems—utilizes semiconductors. Their impact is vast, driving innovation across industries while influencing economies globally. The significance of understanding how this technology evolved cannot be overstated.
In this section, we will explore what semiconductors are and why they are crucial in shaping our technological landscape.
Defining Semiconductors
The term "semiconductor" refers to a class of materials whose electrical conductivity lies between that of a conductor and an insulator. Often made from silicon or germanium, semiconductors can conduct electricity under certain conditions, making them versatile components essential for constructing electronic circuits.
These materials exhibit unique properties:
- Conductivity that can be controlled: Doping, a process where impurities are added, enhances the material's conductivity significantly.
- Response to temperature: Semiconductors can change their conductive properties with temperature variations, making them ideal for temperature-sensitive applications.
Understanding their characteristics allows engineers to devise technology that has revolutionized how we communicate, consume media, and access information. In other words, semiconductors are the unsung heroes behind the curtain, quietly powering the devices that keep us connected.
Historical Overview
The journey of semiconductor technology is intertwined with the evolution of modern electronics. It all began in the early 20th century when scientists started identifying the conductive properties of materials like silicon.
Key milestones in this timeline include:
- 1904: John Ambrose Fleming invented the vacuum tube which laid the foundations for electronic devices.
- 1947: The invention of the transistor by John Bardeen, Walter Brattain, and William Shockley marked a significant turning point, replacing bulky vacuum tubes and setting the stage for the miniaturization of electronic components.
- 1950s-60s: The rise of integrated circuits, driven by Robert Noyce and Jack Kilby, allowed multiple transistors to be fabricated into a single chip, leading to drastic reductions in size and cost of electronics.
- 1970s-2000s: Advancements in microelectronics led to the proliferation of personal computers and the internet, making technology accessible to the masses.
Reflecting back on these developments, it’s clear that semiconductor technology not only transformed products but also fundamentally altered society. This historical perspective islandifies how each breakthrough set the stage for subsequent innovations, underscoring the profound role of semiconductors in driving progress throughout the decades.
Fundamental Properties of Semiconductors
Understanding the fundamental properties of semiconductors is crucial for illuminating their role in modern electronics. These properties not only dictate how semiconductors behave under various conditions but also inform the design and development of countless devices that form the backbone of today’s technology. From smartphones to supercomputers, these elements contribute significantly to performance, efficiency, and versatility in applications. Here, we will dive into three key areas: electrical conductivity, band theory, and the distinctions between intrinsic and extrinsic semiconductors.
Electrical Conductivity
Electrical conductivity is perhaps the most pivotal property of semiconductors. Unlike conductors that allow electricity to flow freely or insulators that resist it, semiconductors fall in between. This trait earns them a unique position in the tech landscape. The level of conductivity can be fine-tuned through various methods, including temperature alterations and doping.
When examining the conductivity of semiconductors, it's essential to understand that their ability to conduct electricity depends on the movement of charge carriers, which are typically electrons and holes. At higher temperatures, the thermal energy enables more electrons to jump from the valence band to the conduction band, facilitating current flow. Consequently, as temperature rises, so does conductivity, resulting in what is known as negative temperature coefficient behavior.
Moreover, the process of doping introduces impurities into the semiconductor to modify its intrinsic properties. Doping with elements like phosphorus can add extra electrons, thereby creating an n-type semiconductor, whereas doping with boron can create p-type semiconductors by generating holes. This ability to tailor conductivity is what makes semiconductors invaluable in designing components like transistors, diodes, and integrated circuits.
Band Theory
Band theory provides a theoretical framework that explains how semiconductors conduct electricity. In essence, it describes the energy bands in solids, focusing on the valence and conduction bands. The spacing between these bands plays a critical role in determining the electrical properties of the material.
In a semiconductor, the band gap is the energy difference between the valence band, which is filled with electrons, and the conduction band, which is where electrons can move freely to conduct electricity. Typically, the band gap for semiconductors is small enough that at room temperature, some electrons gain enough energy to leap into the conduction band, creating charge carriers and allowing electrical conduction.
The band gap also influences the semiconductor's behavior under different lighting conditions. For instance, materials like silicon have a band gap of about 1.1 eV, which is pivotal for applications such as solar cells, where photons provide the energy needed for electrons to transition to the conduction band. Different semiconductors offer various band gaps, allowing for tailored applications across fields ranging from optoelectronics to power generation.
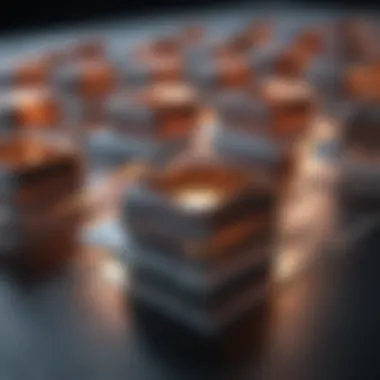
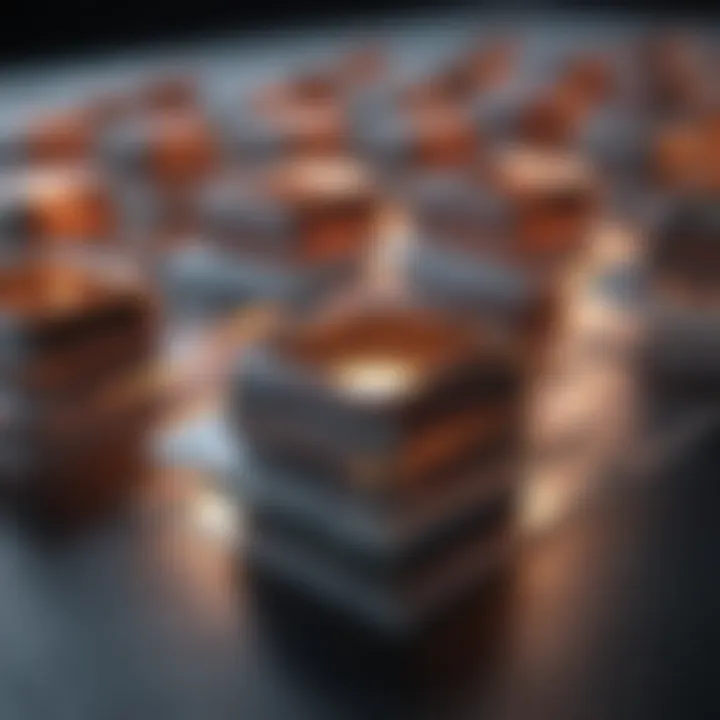
Intrinsic and Extrinsic Semiconductors
When we talk about semiconductors, it is vital to differentiate between intrinsic and extrinsic types. Intrinsic semiconductors, like pure silicon or germanium, possess their electrical properties without any intentional doping. Their conductivity is generally lower than that of extrinsic semiconductors because the number of charge carriers is only determined by the thermal excitation of electrons.
On the other hand, extrinsic semiconductors gain their electrical characteristics from the introduction of dopants. This process significantly enhances the number of available charge carriers, allowing for improved conductivity. As mentioned earlier, n-type semiconductors have extra electrons, while p-type semiconductors have additional holes. This distinction is essential in digital circuits, where semiconductors can act as switches, enabling or blocking the flow of current.
In summary, a profound comprehension of the fundamental properties of semiconductors lays the groundwork for grasping the intricacies of semiconductor technology.
"The world of semiconductors is like a tightly woven tapestry, with each thread representing an essential property contributing to the whole."
Recognizing the interplay between electrical conductivity, band theory, and the two types of semiconductors sets the stage for advancements that redefine modern electronics.
Semiconductor Fabrication Processes
Semiconductor fabrication processes play a crucial role in the development of modern electronics, acting as the backbone of everything from smartphones to supercomputers. These processes ensure that the materials used in the creation of semiconductor devices meet specific performance criteria and can operate efficiently at micro and nano scales. Understanding the intricacies of fabrication is essential, especially when considering how these methods influence device performance, cost, and scalability.
In the fab world, there’s an adage that goes, "You can't build a castle without a strong foundation." This saying holds true as the underlying manufacturing processes must be precise and controlled to produce high-quality semiconductors. The three key aspects of semiconductor fabrication processes are wafer production, lithography techniques, and doping methods.
Wafer Production
Wafer production is the starting point of semiconductor manufacturing. Without a good-quality wafer, even the best processes and technologies won't yield effective results. Typically, silicon wafers are formed from a single crystal structure, which is crucial for electrical properties. The purity and structural integrity of these wafers directly impact device performance.
The main steps in wafer production include:
- Crystal Growth: In a process called the Czochralski method, a single crystal of silicon is grown by melting high-purity silicon and pulling a seed crystal upward through the molten silicon.
- Wafer Slicing: The long cylinder of silicon, known as a boule, is sliced into thin wafers using diamond blades, ensuring minimal waste and maximum precision.
- Wafer Polishing: Each slice is polished to achieve a mirror-like surface, which is critical for subsequent photolithography steps.
Quality control is non-negotiable here. Any defects at this stage can ripple throughout the manufacturing pipeline, leading to poor yields down the line.
Lithography Techniques
Lithography is where the magic happens; it allows manufacturers to transfer intricate patterns onto the wafer surface. This stage is akin to drawing blueprints for every tiny component of a semiconductor device. Precision is everything, as even a minor misalignment can derail the entire process.
The popular lithography methods include:
- Photolithography: This uses light to transfer patterns from a mask onto the photoresist-coated wafer, followed by chemical etching to carve out the designs.
- Extreme Ultraviolet Lithography (EUV): A more advanced technique that utilizes shorter wavelengths to create smaller features, pushing the boundaries of miniaturization.
- Interference Lithography: This employs the interference of light waves to create patterns, often used for producing photonic devices.
Advancements in lithography continuously evolve to meet the demand for smaller, faster, and more efficient chips. However, the cost involved in these cutting-edge techniques can strain budgets, leading to ongoing discussions about return-on-investment for innovation.
Doping Methods
Once the patterns are etched, doping comes next. Doping introduces specific impurities into the silicon wafer to alter its electrical properties, tailoring it for various applications. The basic premise here is to enhance conductivity, switching silicon from an "insulator" to a "conductor."
The most commonly used doping techniques include:
- Diffusion Doping: This involves heating the wafer in a container with the dopant gas, allowing the atoms to settle into the silicon lattice.
- Ion Implantation: This method fires ions of the dopant material directly into the wafer at high speeds. It offers better control and uniformity over the doping process.
Understanding these methods is vital, as improper doping can compromise the electrical properties of the semiconductor, leading to failure in applications. As devices become smaller and more complex, achieving precise control over the doping process becomes ever more critical.
"The role of fabrication processes goes beyond just assembly; it shapes the future of technology itself."
The Role of Nanotechnology in Semiconductors
Nanotechnology has made a significant mark in the field of semiconductor technology, altering not only how semiconductors are manufactured but also their overall performance. At its crux, nanotechnology involves manipulating materials at the atomic or molecular level, leading to innovations that were once deemed impossible. This section delves into the vital contributions of nanotechnology to semiconductors, particularly its advancements in nanoscale materials and quantum dots. Understanding these elements is essential, as they pave the way for the next generation of electronic devices and systems.
Nanoscale Materials
With the miniaturization of technology spiraling forward, the role of nanoscale materials has become increasingly important. These materials, often in the range of 1 to 100 nanometers, exhibit unique properties that differ markedly from their bulk counterparts. One remarkable aspect is their increased surface area, which enhances chemical reactivity. This is pivotal in semiconductor applications since it can lead to more efficient energy conversion.
At the nanoscale, materials such as graphene, carbon nanotubes, and nano-silicon offer alternative pathways in improving electronic properties. For instance, graphene has been lauded for its exceptional electrical conductivity and mechanical strength, making it an ideal candidate for next-gen transistors.
Some key benefits of utilizing nanoscale materials include:
- Improved Performance: Devices can operate faster and consume less energy.
- Greater Flexibility: The properties of these materials can be tuned to meet specific needs.
- Cost Reductions: Smaller materials can lead to less waste during production, enhancing cost-effectiveness.
However, one must consider certain challenges. The handling and characterization of nanoscale materials often require specialized techniques and tools, raising barriers for some manufacturers.
Quantum Dots
Quantum dots represent a fascinating area within nanotechnology, particularly relevant to the semiconductor industry. These tiny semiconductor particles, measuring merely a few nanometers in size, exhibit quantum mechanical phenomena, which significantly alters how they interact with light and electricity.
In applications, quantum dots are instrumental in displays, solar cells, and biological imaging. Their unique property of tunable band gaps allows for the emission of various colors, making them a strong candidate for use in high-efficiency display technologies such as QLED TVs.
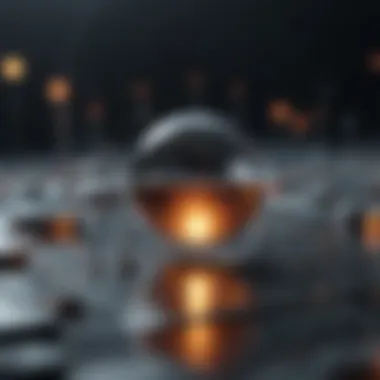
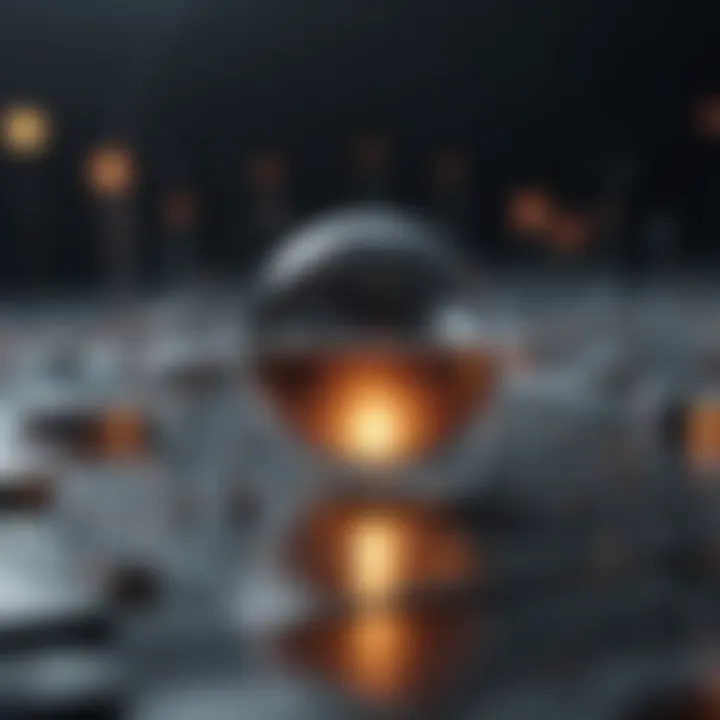
The advantages that quantum dots bring along include:
- High Efficiency: They can convert light with minimal energy loss, improving device efficiency.
- Versatility: Their size allows for customization across a variety of electronic and optical applications.
- Stability: Compared to traditional fluorescent dyes, quantum dots tend to be more stable and longer-lasting.
Though promising, working with quantum dots presents hurdles too. For instance, the materials used in production are often costly, and ethical considerations arise regarding the environmental impact. Addressing these concerns is crucial as the industry pushes for wider adoption.
In summary, integrating nanotechnology into semiconductor applications not only enhances performance and efficiency but also challenges the industry to innovate responsibly. The promise of nanoscale materials and quantum dots signifies an exciting frontier in semiconductor technology.
Applications of Semiconductor Technology
The applications of semiconductor technology are varied and extensive, playing a pivotal role in multiple sectors. Understanding these applications allows us to appreciate the profound influence semiconductors have on current technological advancements and everyday life. In this section, we will delve into their significance in consumer electronics, the automotive industry, and telecommunications, outlining the unique contributions and reflections of semiconductor technology in each.
Consumer Electronics
In the realm of consumer electronics, semiconductors act as the beating heart of various devices. Smartphones, laptops, televisions, and gaming consoles all rely on the intricate circuitry designed with semiconductor materials. Their ability to efficiently handle signals and manage energy consumption makes them indispensable in modern technology. For instance, the processors found in smartphones like the Apple A15 Bionic or Qualcomm Snapdragon 888 exemplify the cutting-edge design that enhances performance while maintaining energy efficiency.
When we consider the features that most consumers cherish, such as high-resolution displays, rapid processing speeds, and compact designs, it becomes evident that these advancements are rooted in semiconductor technology. Furthermore, the integration of features like artificial intelligence for photography or real-time language translation occurs due to the enhancements in semiconductor capabilities.
"The use of semiconductors has revolutionized consumer electronics, giving birth to devices that are not just functional but also intelligent."
Automotive Industry
The automotive industry has also seen a seismic shift thanks to semiconductor technology. The shift towards electric vehicles (EVs), for example, has necessitated advanced semiconductor solutions that manage the power systems crucial for operation. Components like power inverters, battery management systems, and electric drive motors are all driven by semiconductors. Companies like Tesla and General Motors are leading the way, heavily relying on these technologies to push the boundaries of performance and efficiency.
Moreover, modern vehicles equipped with semi-autonomous features employ a complex array of semiconductors to handle everything from navigation systems to collision avoidance sensors. The increasing demand for connectivity, such as 5G integrations, further reflects the importance of semiconductors in automotive design and safety features. They ensure vehicles can communicate seamlessly with external systems, enhancing both safety and the overall driving experience.
Telecommunications
Turning our gaze to telecommunications, we observe that semiconductors form the foundation of network infrastructure. From base stations to routers, the efficiency and speed of data transmission rely on cutting-edge semiconductor technology. The rapid rollout of 5G networks worldwide is a direct result of advancements in semiconductor materials and manufacturing processes. Companies such as Qualcomm have made significant strides in RF (radio frequency) chipsets that enable faster, more reliable connectivity.
Beyond 5G, the cumulative benefits extend to IoT (Internet of Things) applications. As more devices connect to networks, the role of semiconductors in optimizing communication protocols becomes crucial. For instance, smart homes with interconnected appliances depend on semiconductors to facilitate real-time communications across platforms.
In summary, the applications of semiconductor technology span diverse fields, enhancing the functionality and efficiency of products we often take for granted. This dynamic sector continues to evolve, pushing innovation in consumer electronics, transforming the automotive landscape, and spearheading advancements in telecommunications.
Current Challenges in Semiconductor Manufacturing
As the semiconductor industry continues to evolve at a rapid pace, it faces a multifaceted array of challenges that could significantly impact its future. Recognizing these hurdles is crucial, not only for industry leaders but also for policymakers, researchers, and students alike. Addressing these concerns will help in steering the strategic direction of semiconductor technology toward sustainable growth and innovation.
Supply Chain Disruptions
The semiconductor supply chain is intricate, comprising numerous players from raw material suppliers to fabrication facilities and equipment manufacturers. Recent global events have exposed vulnerabilities within this framework. For instance, the COVID-19 pandemic created unprecedented spikes in demand for electronic devices while simultaneously causing material shortages due to factory closures and logistical delays.
Such disruptions underline the need for a more resilient and flexible supply chain.
- Global Interdependence: Countries rely heavily on one another for various components, making it essential to consider geopolitical factors during supply chain planning.
- Stockpiling and Diversification: Companies are now revisiting strategies like stockpiling key materials or diversifying their supplier base to minimize risk.
Even the most advanced fabrication processes can be rendered ineffective if the supply chain behind them is not stable.
Cost Pressures
The demand for advanced semiconductor technologies, like 5G and AI applications, is constantly escalating. However, this comes with increasing costs that can pose a significant hurdle for many manufacturers. The investments required for state-of-the-art fabrication facilities, coupled with the costs of research and development, can create considerable financial strain.
This situation gives rise to several crucial considerations:
- Return on Investment: Companies must assess whether the expenditures on cutting-edge technology will yield favorable returns.
- Competitive Landscape: Those unable to keep pace due to financial limitations risk falling behind competitors who are rapidly adopting new technologies.
The balance between innovation and affordability could determine which players thrive or falter in the coming years.
Technological Obsolescence
In the fast-paced world of semiconductors, technological obsolescence is a persistent worry. As new innovations emerge, older technologies risk becoming irrelevant almost overnight. This is particularly true with the advent of quantum computing and next-gen materials.
Key factors to monitor include:
- Rapid Product Life Cycles: Consumers’ need for efficiency leads to shorter product life cycles, compelling manufacturers to frequently upgrade their technology.
- Investment in New Technologies: Companies need to allocate resources not just for current technologies, but also for researching future advancements.
For players in the semiconductor arena, staying ahead of obsolescence is not just advantageous; it is essential for sustained success.
Sustainability in Semiconductor Production
As the world increasingly shifts towards more responsible and eco-friendly practices, sustainability in semiconductor production transpires as a pivotal issue. The semiconductor industry, pivotal to modern technology, is facing intense scrutiny regarding its environmental footprint. Understanding sustainability in this sector means addressing the balance between innovation and ecological consideration, ensuring the future of technology does not come at the expense of the planet.
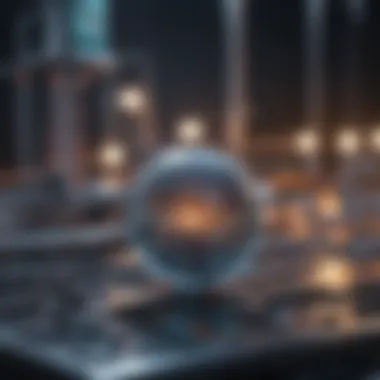
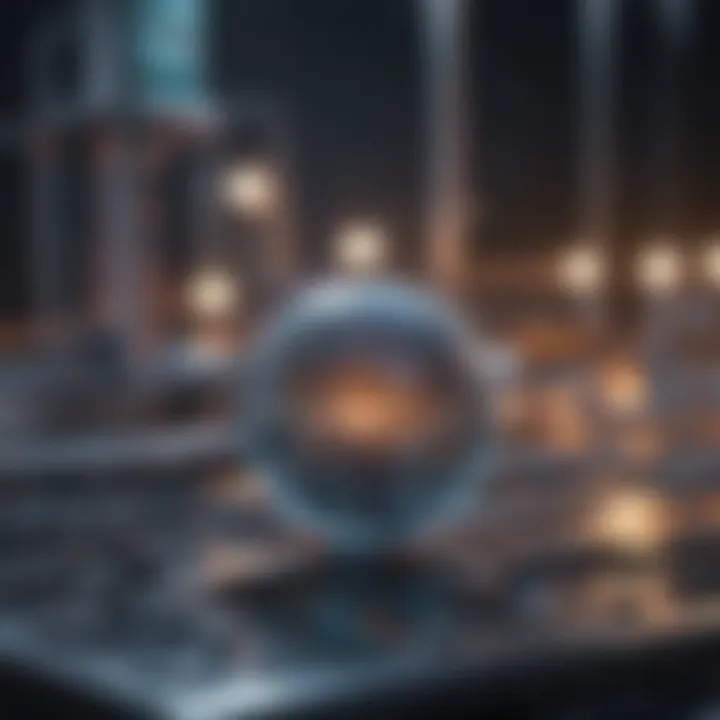
Environmental Impact
The environmental effects of semiconductor fabrication are multi-faceted. The manufacturing processes discharge hazardous chemicals, consume significant amounts of water, and generate considerable waste. For example, the fabrication of a single silicon wafer can require anywhere from 500 to 1500 liters of ultra-pure water. This level of consumption poses serious concerns in regions facing water scarcity. Moreover, waste management becomes a critical aspect since many materials are toxic and require specialized disposal.
In addition, the production of chips often involves greenhouse gas emissions contributing to climate change. With semiconductor companies producing millions of devices each year, the cumulative impact is not negligible. The industry is under pressure to adopt cleaner technologies and methodologies, including the use of renewable energy sources in manufacturing operations. Many manufacturers are beginning to transition to solar and wind energy, a positive step in mitigating their carbon footprint.
"Sustainability should not be an afterthought in semiconductor production; it’s integral to the future of the industry."
Recycling Efforts
Recycling efforts in the semiconductor sector are gaining momentum. Given the high value of materials used, like silicon, germanium, and rare metals, many companies are finding it profitable to reclaim these resources rather than losing them in landfills.
- Material Recovery: Businesses are now employing closed-loop recycling systems that allow the recovery of materials. This process not only conserves resources but also reduces the need for raw material extraction, curtailing environmental degradation.
- Innovative Recycling Programs: Various initiatives exist aiming to recycle old semiconductor devices. For instance, using advanced chemical processes, companies can extract valuable materials from discarded electronics, which helps streamline the supply chain and addresses waste management concerns effectively.
- Regulatory and Industry Standards: New regulations are popping up worldwide, setting strict guidelines for electronic waste disposal and encouraging recycling initiatives. Certification programs are emerging that help consumers identify products made from recycled materials, promoting a circular economy.
In summary, the sustainability narrative in semiconductor production is not solely about reducing negative impacts. It is about embracing a holistic approach that seeks to innovate while also honoring the commitment to the environment. By focusing on these efforts, the semiconductor industry strives towards a more sustainable and responsible future.
Future Trends in Semiconductor Technology
In the rapidly evolving landscape of technology, semiconductor innovation stands at the forefront. Its growth shapes everything from tiny gadgets in our pockets to gigantic data centers that power the internet. Understanding future trends in semiconductor technology is not just for enthusiasts; it's vital for students, researchers, and professionals who want to stay ahead in their fields. The convergence of several factors, including the drive for efficiency, the demand for high performance, and the integration of artificial intelligence, is pushing the semiconductor industry toward exciting new horizons.
Emerging Materials
One of the most compelling trends emerges in the realm of materials science. Traditionally, silicon has dominated the semiconductor landscape, but new contenders are beginning to challenge its throne. Materials like gallium nitride and graphene are gaining ground due to their superior properties, such as higher electron mobility and thermal conductivity. Gallium nitride (GaN) is particularly notable in power electronics, allowing for smaller, more efficient devices that generate less heat.
Meanwhile, researchers are exploring two-dimensional materials, like transition metal dichalcogenides, offering properties conducive to flexible electronics.
Some key points concerning these emerging materials include:
- Efficiency: These new materials potentially enable devices to operate at higher efficiencies compared to traditional silicon-based options, impacting everything from nanotechnology to renewable energy.
- Versatility: They are adaptable for various applications, particularly in wearable technology and the Internet of Things (IoT).
- Environmental Constraints: There is a push for more sustainable materials that minimize harmful waste, addressing environmental concerns within semiconductor production.
This shift not only opens doors for innovative applications but also drives the industry toward a greener, smarter future.
Integration with AI and Machine Learning
The interplay between semiconductors and artificial intelligence (AI) is becoming increasingly significant. As data generation skyrockets, so does the need for efficient processing. AI and machine learning algorithms require exceptional computational power, creating a bustling demand for specialized semiconductor solutions.
A few essential points to consider:
- Neuromorphic Computing: The emergence of neuromorphic chips mimics the human brain's architecture to process information more efficiently. This new approach can reduce power consumption and increase speed, benefiting applications in robotics and autonomous systems.
- Edge Computing: With the rise of IoT devices, edge computing models are gaining traction. Semiconductors designed for edge processing are being optimized to filter, analyze, and react to data in real-time without the burden of cloud processing.
- Customization: There’s an increasing demand for customized processors, such as application-specific integrated circuits (ASICs), tailored to specific AI tasks, enabling improved performance over general-purpose chips.
This integration underscores a larger narrative where computational strategies are evolving to support more advanced AI capabilities, intertwining their future trajectories.
Advances in Quantum Computing
Quantum computing holds its own promise as an area of future semiconductor development. With the potential to outperform classical computers, quantum machines rely on the manipulation of qubits, significantly challenging current semiconductor technologies.
Key developments includes:
- Superconducting Qubits: Research is underway to create stable superconducting qubits through advanced semiconductor processes. These advancements could lead to unprecedented breakthroughs in computational power.
- Cryogenic Computing: The requirements for quantum computing operate at very low temperatures. Innovations in semiconductor materials that can handle these conditions will be pivotal in the next phase of quantum exploration.
- Quantum Algorithms: With emerging quantum algorithms implicating the architecture and designs of semiconductors, it necessitates a rethink of current manufacturing techniques.
This exploration into quantum technology is still unfolding. Many professionals recognize that mastering these components will be essential for harnessing the next era of technological advancements.
As we look toward the future, semiconductor technology will inevitably intertwine with advancements in materials, AI, and quantum computing, shaping a tech-savvy world of tomorrow.
"The complexity of the semiconductor industry mirrors the intricacies of our society; to thrive, adaptation and innovation must go hand in hand."
Embarking on this journey, students, researchers, and professionals are encouraged to keep abreast of these trends, as they will undoubtedly influence various sectors, including healthcare, transportation, and communications.
End
The conclusion of this article highlights the integral role of semiconductor technology in shaping modern society. This technology is not merely a technical marvel; it’s the backbone of countless devices and systems that define contemporary living. A thorough understanding of its evolution, applications, and future possibilities can not only enhance one’s academic or professional prowess but also foster informed decisions in an increasingly tech-driven world.
Summary of Key Points
Throughout this discussion, several key points emerged that underscore the significance of semiconductors:
- Historical Context: The development of semiconductor technology from its early days to present advances reflects an evolution influenced by societal needs and scientific discoveries.
- Fundamental Properties: Understanding electrical conductivity, band theory, and the difference between intrinsic and extrinsic semiconductors is crucial for recognizing how these materials function in various applications.
- Fabrication Techniques: Processes like wafer production and doping methods are essential for creating the semiconductors that power electronic devices.
- Challenges: Current industry hurdles including supply chain disruptions and technological obsolescence highlight the need for strategic planning and innovation.
- Sustainability: As the industry faces environmental scrutiny, recycling efforts and potential shifts to greener materials are critical for sustainable growth.
- Future Trends: The integration of AI and quantum computing with semiconductor technology indicates not only growth but also a shift in how tech might evolve, foreshadowing transformative impacts on various sectors.
"The path ahead for semiconductors is replete with potential, but also fraught with challenges that require deft navigation."
The Path Forward
Looking ahead, the semiconductor industry is positioned to undergo profound changes, influenced by both technological advancements and environmental imperatives. Companies need to adapt to a rapidly evolving landscape, defining strategies that embrace sustainability while also driving innovation.
- Investing in Research: Ongoing research into new materials, such as transition metal dichalcogenides or organic semiconductors, could result in breakthrough applications, optimizing performance while minimizing environmental impact.
- Strengthening Supply Chains: Diversifying supply sources and enhancing logistical frameworks can mitigate the risk of disruptions. The crisis during the early stages of the pandemic illustrated how fragile these chains can be.
- Collaboration and Alliances: Partnerships among academic institutions, industries, and governments can foster knowledge sharing, which is essential for tackling complex challenges.
- Embracing AI: Utilizing artificial intelligence not only in semiconductor design but also in manufacturing processes can significantly improve efficiency and productivity.
In summary, while the road ahead is dotted with complexities, it also promises remarkable opportunities. With the right strategies in place, stakeholders can leverage these trends, ensuring that semiconductor technology continues to thrive and innovate for future generations.