Hydrogen Storage Methods: Comprehensive Analysis
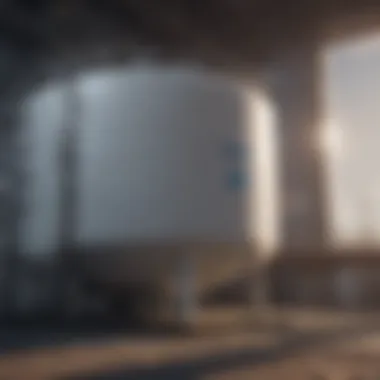
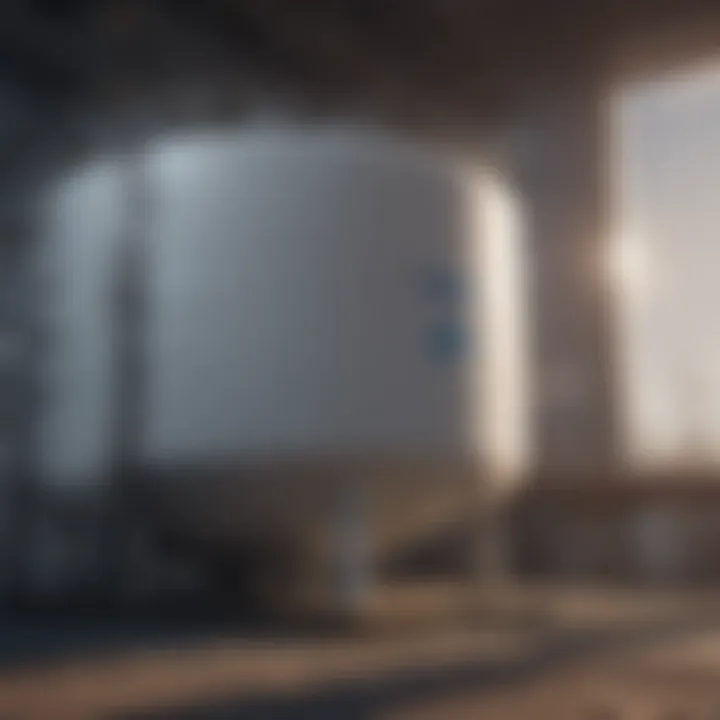
Intro
Hydrogen stands out as a potential game-changer in the quest for sustainable energy solutions. Its versatility and abundance make it an attractive option for various applications, from powering vehicles to serving as an energy storage medium. However, the practical deployment of hydrogen relies heavily on effective storage methods. The storage of hydrogen is a critical aspect of its utilization, as it must be stored safely and efficiently to facilitate transport and usage.
The various methods for hydrogen storage range widely in terms of technology, materials, and implementation strategies. Each method presents unique advantages and challenges, influencing their commercial viability and environmental impact. In this article, we will scrutinize established methods like compressed gas and liquid hydrogen storage. We will also consider emerging technologies including metal hydrides and chemical hydrogen storage.
In exploring these topics, the objective is to provide insight into the efficiencies, challenges, and future prospects of each method. We will assess how these techniques align with broader energy systems and sustainability efforts, offering readers a comprehensive understanding of the evolving landscape of hydrogen storage.
Preface to Hydrogen Storage
Hydrogen has emerged as a crucial player in the transition to sustainable energy systems. This is due to its potential for reducing reliance on fossil fuels and lowering greenhouse gas emissions. The quest for effective hydrogen storage solutions is therefore important in maximizing the efficiency of hydrogen as an energy carrier. Storage methods can determine the viability of hydrogen technologies in various applications, from transportation to electricity generation. This topic deserves comprehensive exploration.
Importance of Hydrogen in Energy Systems
The role of hydrogen in modern energy systems is multifaceted. First, hydrogen can serve as a clean fuel with the capability to produce water as the only by-product during combustion. This positions it as a crucial element in efforts to combat climate change. Furthermore, hydrogen can store energy generated from renewable sources such as solar and wind, which are often intermittent.
- Versatility: Hydrogen's versatility is in its applications; from serving as a fuel in fuel cells to being utilized in various industries, including refining and ammonia production. This adaptability allows hydrogen to fit into multiple facets of energy systems.
- Grid Stability: Hydrogen storage can enhance grid stability by absorbing excess energy during peak production times and releasing energy during shortages.
- Decarbonization: Countries around the world are focusing on hydrogen to help achieve their decarbonization targets. Hydrogen can replace fossil fuels in various sectors, thus reducing overall carbon footprints.
Overview of Hydrogen Storage Challenges
Despite its potential, hydrogen storage faces multiple challenges. The first challenge is energy density. Hydrogen, when stored as a gas, has a low volumetric energy density at ambient conditions, which makes storage space inefficient.
- Material Limitations: Existing materials for hydrogen storage often do not meet the performance requirements necessary for practical applications. Advances in materials science are critical to finding more effective and efficient storage solutions.
- Safety Concerns: Hydrogen is highly flammable and poses safety risks during storage and transportation. Hence, developing safe storage protocols and technologies is essential.
- Economic Factors: High costs associated with the development and implementation of hydrogen storage technologies hinder widespread adoption. This includes initial capital investments and ongoing operational expenses.
Understanding these challenges is crucial for developing future hydrogen storage technologies aimed at promoting greater efficiency and widespread adoption in energy systems.
Types of Hydrogen Storage Methods
Understanding the various hydrogen storage methods is crucial for advancing hydrogen technology in energy systems. Hydrogen storage allows for effective energy management and solidifies hydrogen's role as an essential element in renewable energy resources. Each storage method offers unique benefits and considerations, influencing its suitability for specific applications.
Compressed Gas Storage
Basics of Compressed Hydrogen
Compressed hydrogen storage involves storing hydrogen gas under high pressure, typically between 350 to 700 bar. This method is widely used due to its simplicity and efficiency in transitioning hydrogen from one location to another. The key characteristic of compressed hydrogen is its ability to maintain gaseous state at higher pressures, which leads to a significant reduction in the volume required for storage.
The main advantage of this method is its scalability, allowing for various tank sizes suitable for different uses. However, the high-pressure nature also introduces potential containment challenges.
Advantages and Limitations
The advantages of compressed gas storage include its relatively low cost and high energy density, making it an appealing option for both industrial and commercial applications. It can discharge gas rapidly, which is beneficial for fuel cell vehicles. However, limitations also exist. The energy required to compress hydrogen is substantial, necessitating efficiency considerations. Safety concerns related to high-pressure systems must also be managed effectively.
Applications in Industry
Compressed gas storage finds application across industries, particularly in fuel cell technologies and various manufacturing sectors. Its ability to deliver hydrogen on-demand aligns with the needs of industrial processes. Additionally, it enables transportation solutions for hydrogen fuel cells in vehicles. Despite its broader acceptance, the challenge lies in ensuring the reliability of the pressurized containers and systems in diverse operational environments.
Liquid Hydrogen Storage
Condensation Methods
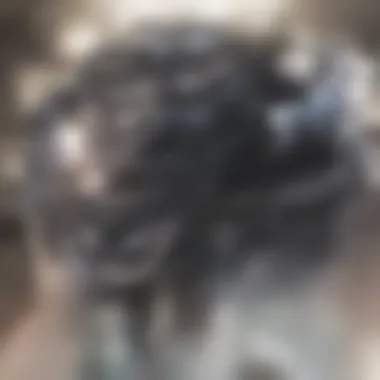
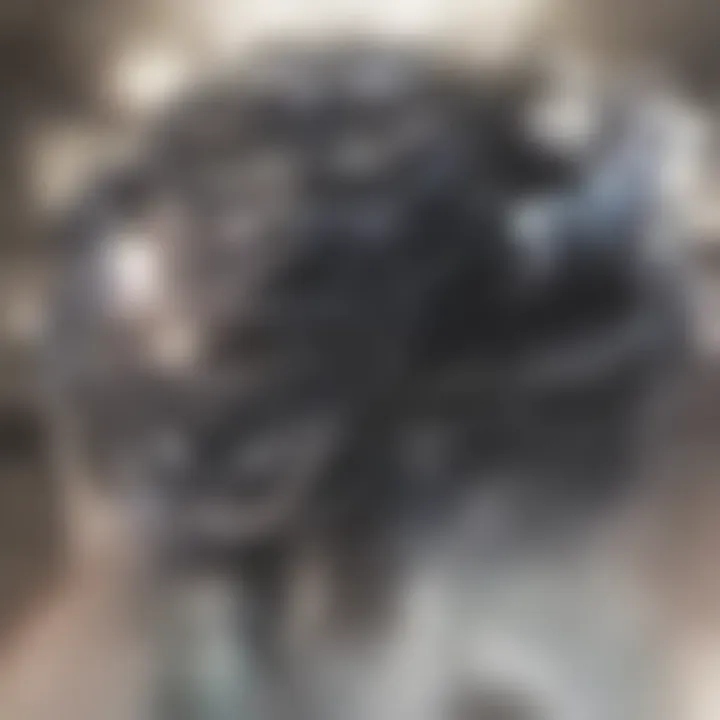
Liquid hydrogen storage necessitates cooling the gas to extremely low temperatures, about -253°C. This process condenses hydrogen into a liquid state, significantly increasing its density. The main benefit of this method is the reduction in volume compared to gaseous storage, which is critical for applications requiring high-capacity storage. Nonetheless, the energy consumption for liquefaction is a considerable drawback.
Thermal Efficiency
Thermal efficiency in liquid hydrogen storage relates to the energy expended in cooling hydrogen to its liquid state and maintaining that state during storage. Techniques to improve thermal management are critical, as any temperature increase can lead to gaseous hydrogen escaping. Higher efficiency measures are essential for minimizing energy losses in the cycle.
Safety Considerations
Safety in liquid hydrogen storage involves addressing the flammability and volatility of hydrogen. Proper insulation and material selections are vital to prevent leaks and manage cryogenic temperatures. Handling protocols need implementation to ensure operator safety, as liquid hydrogen poses distinct risks compared to other fuel storage methods.
Metal Hydride Storage
Mechanism of Metal Hydrides
Metal hydride storage relies on chemical reactions between hydrogen and metal alloys, allowing hydrogen to be absorbed and stored in a solid state. This method typically offers a higher volumetric density than gaseous and liquid hydrogen. The mechanism of absorption is crucial, as it enables the release of hydrogen when needed under controlled conditions. However, the complexity of alloy selection presents challenges in material engineering.
Material Selection
Material selection is a significant factor determining the efficiency and effectiveness of metal hydride systems. Appropriate alloys must be identified based on their hydrogen absorption capacity, kinetics, and thermal stability. While metal hydrides can provide safe storage, their costs and performance metrics can vary widely based on material compositions.
Real-World Applications
The applications for metal hydride storage encompass niche areas such as portable hydrogen tanks and backup energy systems. Their efficient hydrogen release under moderate conditions makes them suitable for specific uses like automotive fuel systems. However, challenges relate to the weight of metal hydrides, impacting their viability for high-performance transport applications.
Chemical Hydrogen Storage
Overview of Chemical Processes
Chemical hydrogen storage utilizes various compounds that release hydrogen through chemical reactions. This method allows for higher storage densities than other conventional methods. It is particularly useful in a cyclic process where carriers are easily regenerated, highlighting the important aspect of sustainability in hydrogen storage. It’s imperative to assess the various chemical pathways for efficacy.
Common Chemical Carriers
Common chemical carriers include ammonia, methanol, and formic acid. Each of these carriers has specific advantages, such as improved stability and transport logistics compared to pure hydrogen. These materials provide a more manageable form for storage and distribution; however, they also require careful handling and processing requirements to extract hydrogen efficiently.
Advantages over Other Methods
Chemical hydrogen storage presents compelling advantages in terms of energy density compared to traditional methods. It also addresses some safety concerns posed by gaseous or liquid hydrogen. However, the complexity of chemical processes can lead to additional costs and energy requirements for conversion.
Adsorption-Based Storage
Principles of Adsorption
Adsorption-based storage operates on the principle of molecules adhering to solid surfaces, allowing for the retention of hydrogen in a compact form. Unlike other methods, this technique can offer potential efficiencies at lower pressures and temperatures. Its capacity to utilize porous materials makes it an attractive area of research. The key challenge lies in maximizing surface area utilization.
Material Research and Development
Continuing research into adsorbent materials is fundamental for optimizing storage capabilities. Researchers work on developing materials such as graphene and carbon nanotubes, which hold promise for higher adsorption capacities. However, material costs and production scalability are ongoing concerns, needing innovative solutions to bring these advancements to fruition.
Potential Applications
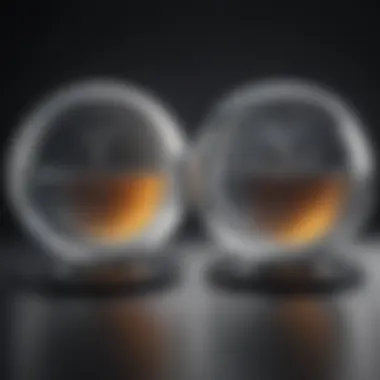
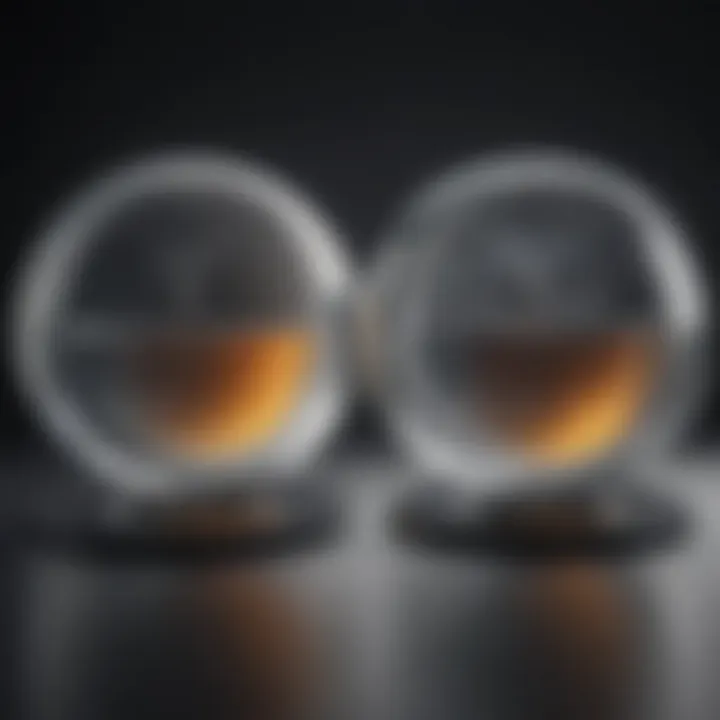
Potential applications for adsorption-based storage include lightweight storage solutions for vehicles and portable energy systems. The unique ability to operate effectively at low energy requirements gives this method an edge in future energy applications. As advancements in materials continue, wider adoption in various sectors appears probable, although market readiness is contingent on successful R&D outcomes.
Material Innovations for Hydrogen Storage
Material innovations play a crucial role in advancing hydrogen storage technologies. They improve efficiency and safety while enabling higher energy densities, which is essential for practical applications of hydrogen as an energy carrier. The constant evolution in material sciences opens new avenues for storing hydrogen, pushing the boundaries of current technologies. This section explores some of the latest advances and focuses on nanomaterials and organic hydrogen storage materials.
Nanomaterials in Hydrogen Storage
Types of Nanomaterials
Nanomaterials are a subclass of materials with structures on the nanometer scale. Their unique properties, such as high surface area and tunable chemical properties, make them an excellent choice for hydrogen storage. Common types include carbon nanotubes, metal-organic frameworks, and graphene. Each of these materials offers distinct advantages like increased adsorption capacity and better kinetics in hydrogen absorption and release. The ability to tailor their properties enhances their applicability, giving them an edge over traditional bulk materials.
Benefits of Using Nanotechnology
The use of nanotechnology in hydrogen storage brings several benefits. One significant aspect is the enhanced efficiency of hydrogen absorption due to increased surface area. This leads to faster kinetics and higher storage capacity, which are vital for industrial applications. Moreover, nanomaterials are lightweight, which is a critical consideration for mobile applications. However, it's important to note the potential scalability issues in manufacturing nanomaterials, as well as potential environmental impacts that require careful evaluation.
Case Studies
Several case studies illustrate the effectiveness of nanomaterials in hydrogen storage systems. For instance, research on carbon nanotubes has shown promising results in terms of hydrogen uptake under moderate pressures and temperatures. Additionally, metal-organic frameworks are being explored for their remarkable hydrogen storage capabilities at ambient conditions. While these materials show potential, challenges remain in terms of cost-effectiveness and long-term stability in real-world applications.
Organic Hydrogen Storage Materials
Overview of Organic Materials
Organic hydrogen storage materials utilize chemical compounds that can reversibly store hydrogen. These materials, often derived from sustainable sources, offer the potential for greener hydrogen production and storage methods. The light weight of organic materials enables better portability and integration into existing infrastructure. Key examples include ammonia, formic acid, and various organometallic compounds, each providing different pathways for effective hydrogen storage.
Advantages and Limitations
The use of organic materials for hydrogen storage comes with clear advantages, such as lower costs and environmental friendliness. They can often be stored and transported under milder conditions compared to gaseous or liquid hydrogen. However, limitations exist, including often lower hydrogen density compared to more traditional storage methods. Stability and safety also raise concerns, especially with certain chemicals that might be toxic or hazardous, necessitating rigorous safety evaluations.
Emerging Trends
Emerging trends in organic hydrogen storage materials indicate a shift towards more advanced formulations and hybrid systems. Researchers are exploring hybrid systems that combine organic compounds with nanomaterials to enhance performance. Interest in biobased materials is also growing, reflecting a sustainable approach to hydrogen storage. Nevertheless, the challenge lies in optimizing these new materials for commercial viability, ensuring that they meet both cost and performance benchmarks.
"Advances in materials for hydrogen storage are critical to overcome current limitations in storage efficiency and safety, paving the way for broader hydrogen adoption in energy technologies."
These material innovations are vital for improving hydrogen storage systems, making them an important focus in contemporary research.
Safety and Environmental Considerations
The safe storage and handling of hydrogen are crucial as the demand for this fuel increases. Hydrogen is a lightweight and highly flammable gas, which presents unique challenges in both its storage and transportation. Therefore, understanding safety protocols and assessing the environmental impacts of hydrogen storage systems are vital for their successful integration into energy systems.
Safety must be prioritized to prevent accidents. This is particularly important given the increasing use of hydrogen in various sectors, such as transport and energy. Ensuring that hydrogen storage processes adhere to strict safety standards reduces risks and promotes public confidence. Moreover, analyzing the environmental impact helps identify best practices that align with sustainability goals, making this a significant aspect of hydrogen utilization in modern energy systems.
Safety Protocols for Hydrogen Storage
Robust safety protocols are essential for managing hydrogen effectively. Key protocols include:
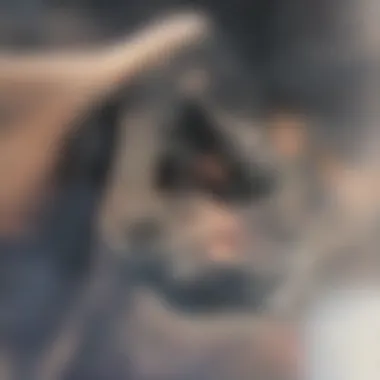
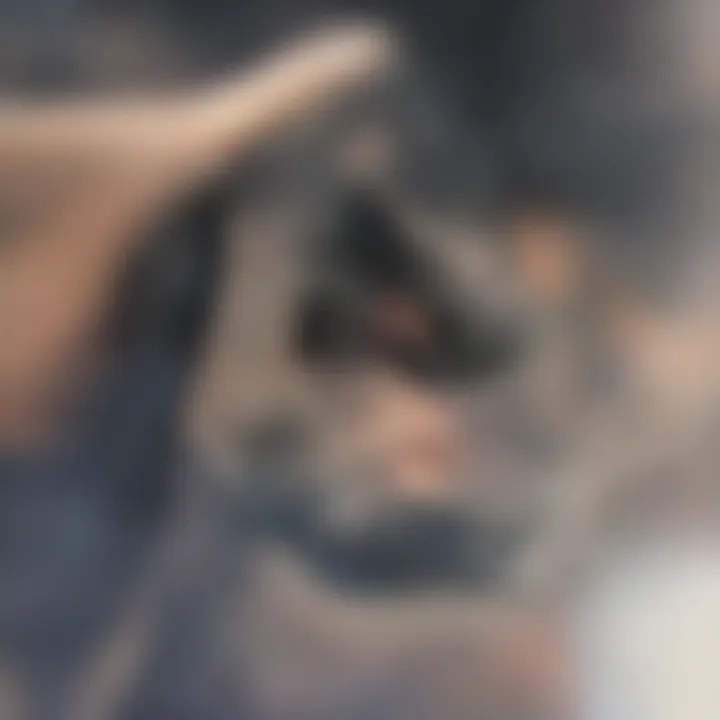
- Leak Detection: Continuous monitoring systems to detect leaks are critical. The use of infrared sensors can identify hydrogen gas releases early, enabling timely intervention.
- Pressure Regulation: Since hydrogen is stored under high pressure, appropriate pressure relief devices must be used to manage any unexpected surges, minimizing the risk of explosions.
- Training Personnel: Those involved in the handling of hydrogen must be trained in emergency response and management practices to ensure that they understand potential hazards.
- Material Selection: The choice of materials for storage tanks and pipelines must withstand hydrogen's properties. This includes using materials that are resistant to hydrogen embrittlement, which can weaken structures over time.
By implementing these protocols, facilities can minimize risks associated with hydrogen storage, ensuring that operations are both safe and efficient.
Environmental Impact of Hydrogen Storage Systems
The environmental implications of hydrogen storage methods warrant careful examination. While hydrogen itself is a clean fuel with water as its only byproduct when burned, the processes involved in its storage can have various environmental effects. Key points of consideration include:
- Life Cycle Analysis: Assessing hydrogen storage through a life cycle approach helps understand the environmental impact from production to disposal. This includes examining energy consumption and emissions related to each storage method.
- Resource Utilization: The manufacturing of storage vessels and systems often necessitates the use of raw materials like metals and composites. Evaluating the sustainability of these materials is crucial in assessing the overall environmental footprint.
- Potential for Pollution: If hydrogen is produced from fossil fuels, this can lead to significant carbon emissions. It is important to promote green hydrogen, generated from renewable energy sources like wind and solar, to mitigate this impact.
The environmental benefits of hydrogen storage are maximized when coupled with renewable energy systems, significantly aiding the transition to a sustainable energy framework.
Understanding these aspects allows developers and policymakers to address challenges effectively while enhancing the role of hydrogen in achieving energy sustainability.
Future Trends in Hydrogen Storage
As the world moves toward a more sustainable and low-carbon future, the role of hydrogen becomes increasingly significant. Understanding the future trends in hydrogen storage is crucial for grasping how this energy carrier can support energy transitions. Innovations in technology and the integration of hydrogen with renewable energy sources present unique opportunities and challenges. This section delves into emerging technological advancements and the synergy between hydrogen and renewable energy, providing a perspective on how these trends shape the energy landscape.
Technological Advancements
The field of hydrogen storage is seeing rapid advancements in technology. Researchers are exploring various methods to enhance storage efficiency, safety, and cost-effectiveness. These technological improvements involve novel materials and approaches.
- Advanced Materials: New materials such as solid-state hydrogen storage systems and advanced metal hydrides have been identified as promising alternatives. Researchers are focused on increasing hydrogen absorption capacities while maintaining safe operating conditions.
- Energy Density Enhancements: Methods that increase the volumetric and gravimetric energy density of hydrogen storage systems are critical. Innovations to innovate the architecture of storage systems aim to make them more compact while increasing the stored hydrogen amount.
- Smart Storage Systems: Integration of Internet of Things (IoT) technologies into hydrogen storage systems is another avenue. Smart sensors and data analytics can optimize the management and monitoring of storage, thereby enhancing efficiency.
- Recycling and Sustainability: Future trends also encompass the recycling of materials used in hydrogen storage, minimizing the overall environmental impact. This increases the sustainability aspect, a key consideration in modern energy paradigms.
Advancements in technology are essential to tackle challenges associated with current hydrogen storage methods, making the energy transition feasible.
Integration with Renewable Energy Sources
Integrating hydrogen storage systems with renewable energy sources, such as solar and wind, presents a significant avenue for enhancing energy security. When renewable energy production exceeds demand, excess energy can be converted into hydrogen through electrolysis. This process not only helps in balancing energy supply and demand but also provides a means of long-term energy storage.
Key aspects of this integration include:
- Renewable Hydrogen Production: The use of electrolysis powered by renewables, known as green hydrogen, is pivotal. This reduces the carbon footprint associated with hydrogen production, aligning it more closely with sustainability goals.
- Grid Stability: Hydrogen can potentially stabilize electrical grids. By acting as a buffer, hydrogen storage can absorb excess electricity, helping to manage fluctuations in production from renewable sources.
- Decarbonization of Industries: Incorporating hydrogen into sectors dependent on fossil fuels, like steel manufacturing or heavy transportation, can significantly reduce carbon emissions. Utilizing hydrogen generated from renewable sources creates a closed-loop energy consumption model.
- Infrastructure Development: Realizing this integration requires robust infrastructure improvements. Investments in pipelines, refueling stations, and storage facilities are necessary to support large-scale hydrogen deployment.
The future of hydrogen storage is thus intertwined with advancements in technology and renewed focus on renewable energy integration. This holistic view ensures that hydrogen storage plays its part in achieving a sustainable energy future.
"The synergy between hydrogen and renewable energy holds the potential to transform the global energy landscape, making it essential to track these trends and invest in the technologies that enable them."
Finale
The conclusion of this article serves as a crucial synthesis of the various hydrogen storage methods discussed. The complexity of hydrogen storage requires careful consideration of each technique's distinct advantages and drawbacks. Understanding these nuances is vital for optimizing hydrogen as a clean energy carrier, which is integral to transitioning towards a more sustainable energy landscape.
Summary of Key Insights
In reviewing the key insights from the previous sections, it becomes clear that hydrogen storage is not a one-size-fits-all solution. The methods available, ranging from compressed gas to liquid hydrogen, metal hydrides, chemical storage, and adsorption-based systems, present unique properties suited for different applications.
- Compressed Gas Storage: This method benefits industries needing high volume but poses challenges during transportation.
- Liquid Hydrogen Storage: It offers high energy density but requires extreme temperatures, introducing safety concerns.
- Metal Hydride Storage: Metal hydrides operate at lower pressures but involve complex material selection.
- Chemical Hydrogen Storage: This method provides a viable alternative, with various chemical carriers demonstrating promising results.
- Adsorption-Based Storage: Advances in material development may enhance operational efficiencies in this emerging field.
Overall, these insights indicate that research and development are essential to address the barriers currently facing hydrogen storage technologies.
Implications for Future Research
The implications for future research in hydrogen storage are substantial. Continued investment in technology development is necessary to improve efficiencies, reduce costs, and enhance safety. Researchers should focus on several critical areas:
- Material Innovation: Advancing nanotechnology and organic materials will likely yield breakthroughs in storage capabilities.
- Integration with Renewable Energy: Exploring how hydrogen storage can work seamlessly with solar, wind, and other renewable sources will provide significant benefits.
- Safety Protocol Enhancements: Developing more stringent safety protocols will ensure wider adoption and consumer confidence.
- Policy and Regulation: Supporting policies that promote research funding and frame necessary regulatory measures can foster innovation.