Understanding GFAP Western Blot Techniques and Applications
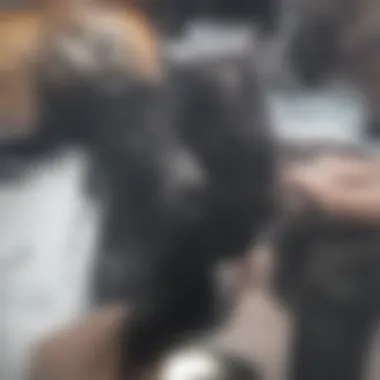
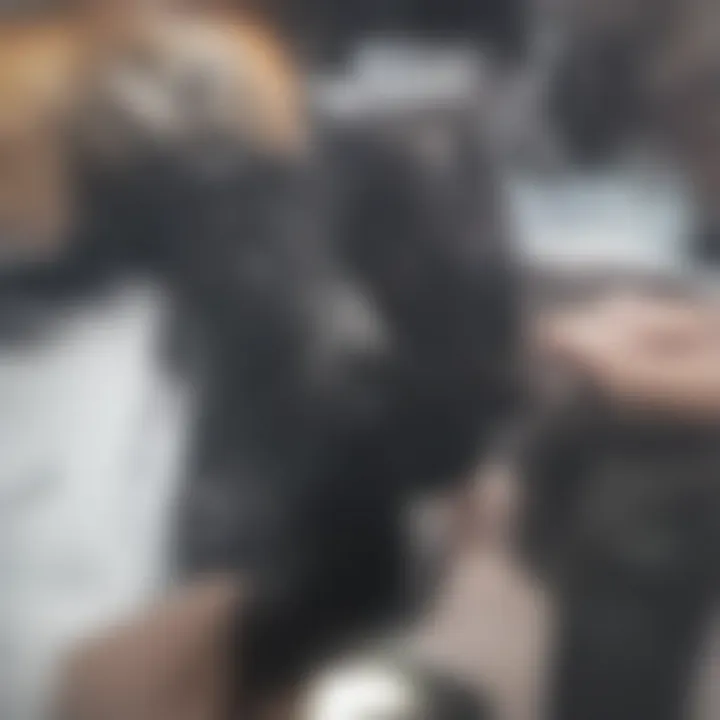
Intro
GFAP, which stands for glial fibrillary acidic protein, is a key protein found in astrocytes, a type of glial cell in the brain and spinal cord. The significance of GFAP lies in its role as a structural protein and as a marker for astrocyte activation. Understanding GFAP levels can provide insights into various neurological conditions, marking it as a pivotal component of neurobiological studies.
Western blotting is one of the techniques used to analyze GFAP. This method allows researchers to detect specific proteins in a sample, providing valuable data on their quantity and the condition of the tissue from which they were drawn. In the field of neuroscience, GFAP Western blot analysis is applied to assess changes in astrocyte activity under different pathological conditions.
Exploring GFAP through Western blotting opens doors to understanding brain pathology, including neurodegenerative diseases, traumatic brain injuries, and more. Its implications are not just theoretical but extend into potential clinical applications, offering diagnostic insights and therapeutic targets. The following sections will dissect the foundational principles of Western blotting, dive into specific protocols for GFAP detection, and discuss the relevance of GFAP in various neurological contexts.
By reviewing these topics, we can appreciate how GFAP Western blot contributes to our comprehension of cerebral functionality and the cellular responses inherent to different neurological disorders.
Prelude to GFAP and Western Blotting
The analysis of glial fibrillary acidic protein (GFAP) via Western blotting serves as a cornerstone technique in modern neurobiology research. Understanding GFAP and its relation to various neurological conditions is crucial for both basic and applied sciences. This section sheds light on the significance of GFAP and details the principles of Western blotting, which make it possible to investigate the roles of astrocytes in the central nervous system.
Overview of GFAP
GFAP is a class of intermediate filament protein that is primarily found in astrocytes, one of the central nervous system's most abundant cell types. Astrocytes play a key role in supporting neurons and maintaining the blood-brain barrier, thus emphasizing the importance of GFAP in cell signaling and neuroprotection. The expression levels of GFAP can increase significantly during reactive astrogliosis, a process critical in response to brain injury or neurodegeneration. This elevation in GFAP levels has made it a potential biomarker for various pathological states, such as neuroinflammation and neurodegenerative diseases.
Notably, the ability to quantify GFAP through techniques like Western blotting enables researchers to explore the intricate cellular response to damage in greater detail. By obtaining precise measurements of GFAP, scientists can gain insights into how astrocytes adapt and modify their functions in various neurological contexts.
Principles of Western Blotting
Western blotting is a well-established method for detecting specific proteins in a complex biological sample. The technique generally involves several key steps: sample preparation, gel electrophoresis, transfer to a membrane, blocking non-specific sites, and probing with antibodies. This method separates proteins based on their size and allows for the identification and quantification of specific proteins, such as GFAP, through the use of antibodies.
The principle underlying Western blotting is that proteins, when subjected to an electric field during gel electrophoresis, migrate according to their molecular weight. Smaller proteins move faster than larger ones, creating a size-based separation. Subsequent transfer to a membrane effectively immobilizes these proteins for analysis. Then, blocking agents are used to prevent non-specific binding, which is essential for accurate detection. Finally, the use of primary and secondary antibodies enables the visualization of target proteins, highlighting the presence and quantity of GFAP in samples.
Furthermore, the versatility of Western blotting allows for the integration of various detection methods, such as chemiluminescence or fluorescence. These techniques further improve sensitivity and specificity, enhancing the utility of GFAP as a reliable biomarker in research settings. As academia continues to explore the implications of astrocyte function in health and disease, understanding both GFAP and the principles of Western blotting is vital.
Importance of GFAP in Neurobiology
GFAP, or glial fibrillary acidic protein, holds significant importance in the realm of neurobiology. Understanding GFAP provides insights into various neurological changes and pathologies, especially those related to astrocyte function. Astrocytes, the most abundant glial cells in the central nervous system, play crucial roles in maintaining homeostasis, providing support to neurons, and responding to injury. Analyzing GFAP levels allows researchers to gauge astrocyte activation and their involvement in disease processes.
There are several reasons why GFAP is critical in neurobiology. Firstly, GFAP is widely recognized as a marker for astrocytes. Its expression level typically rises during brain injury or neurodegeneration, indicating the astrocytes’ response to these events. Monitoring GFAP levels can signal alterations in glial cell function, offering valuable data on the neuroinflammatory state of the brain. Such information is vital for developing therapeutic strategies and for understanding disease progression in patients.
Secondly, GFAP serves a diagnostic function in various neurological conditions. Elevated GFAP levels are associated with disorders such as Alzheimer's disease, multiple sclerosis, and traumatic brain injury. By quantifying GFAP from cerebrospinal fluid or brain tissue samples, researchers can assess the severity of these disorders and evaluate the efficacy of treatments.
In addition, GFAP has potential implications for understanding the relationship between astrocytes and other cell types within the brain microenvironment. For example, investigating the interplay between GFAP and neuronal health could reveal interactions fundamental to brain function and pathology.
Astrocytic contributions to the inflammatory response and recovery process make GFAP a focal point in neurobiology research. As studies continue to uncover the nuances of GFAP’s role in both health and disease, researchers expect to integrate GFAP analyses into broader neurobiological frameworks, aiming to foster a deeper comprehension of brain function and dysfunction.
"GFAP is not just a marker; it is a window into the state of astrocytes and, consequently, brain health."
Role of Astrocytes
Astrocytes, which express GFAP, are multifunctional cells that contribute significantly to the brain's architecture and functionality. They provide structural support, regulate the synaptic environment, and manage neurotransmitter levels. This complex involvement in maintaining neurotransmission makes astrocytes vital players in both normal physiology and pathological conditions.
Their responsiveness to injury demonstrates astrocytes' pivotal roles in the brain's repair mechanisms. The reactive gliosis phenomenon, characterized by increased GFAP expression, reflects astrocyte activation in response to various forms of brain stress. Studying these dynamics sheds light on how astrocytes facilitate recovery but can also lead to maladaptive responses that exacerbate neuronal damage. This duality of their function underscores the importance of GFAP in understanding the neurobiology of diseases.
GFAP as a Biomarker
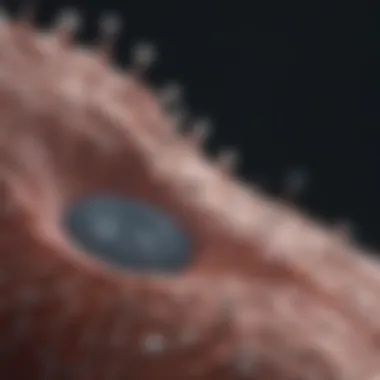
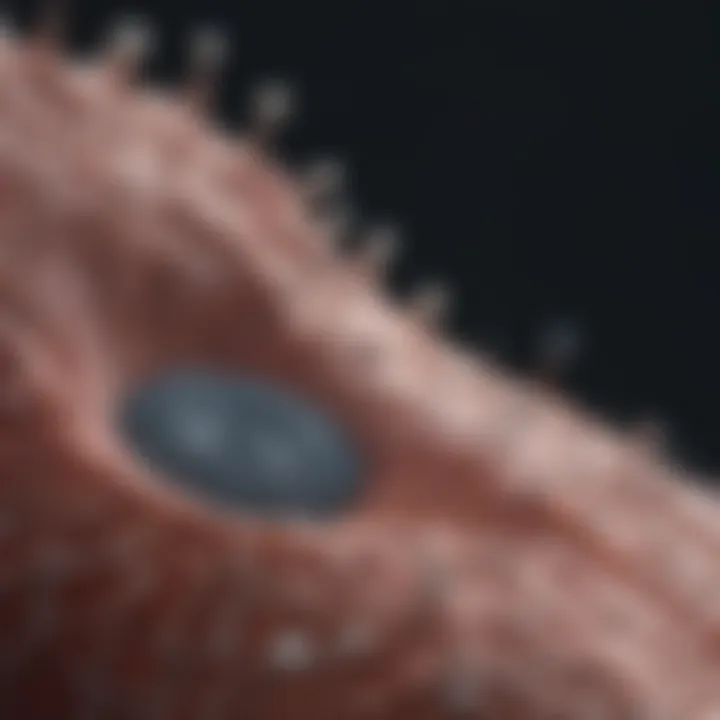
GFAP's role as a biomarker further solidifies its importance in neurobiology. As a marker of astrocyte activation, its levels correlate with the extent of astrocytic response to various neurodegenerative diseases. Clinicians and researchers use GFAP levels to assess brain injury severity and monitor treatment outcomes.
Additionally, elevated GFAP levels in cerebrospinal fluid have been linked to conditions like multiple sclerosis and traumatic brain injury, making it a reliable indicator for diagnosing these diseases. The ability to measure GFAP provides a non-invasive method to track disease progression over time, informing treatment decisions and enhancing patient care.
Methodology of GFAP Western Blotting
The methodology of GFAP Western blotting is essential for researchers delving into neurobiology. It outlines the steps needed to accurately detect GFAP, a key protein in astrocyte function. A thorough understanding of this methodology enables reproducible results. Each step can significantly affect the outcome.
Sample Preparation
Sample preparation is a crucial first step in the Western blotting process. To obtain reliable data, researchers must ensure that the samples are accurately collected and processed. Tissue samples should be homogenized in a suitable buffer to extract proteins effectively. The use of protease and phosphatase inhibitors helps to prevent degradation. Important factors include temperature control and avoiding repeated freeze-thaw cycles, as these can compromise protein integrity. Proper quantification of protein concentration also needs to be done using assays like BCA or Bradford.
Gel Electrophoresis Techniques
Once sample preparation is complete, gel electrophoresis is performed to separate proteins by size. Polyacrylamide gels are commonly used for this purpose. The percentage of the gel must be chosen according to the size of GFAP. For instance, a 10% gel is typically appropriate for detecting GFAP. Loading equal amounts of protein is vital to ensure normalization across samples. Electrophoresis should be conducted under controlled voltage and temperature, with adequate running time to achieve resolution.
Transfer to Membrane
The transfer of proteins from the gel to a membrane is the next critical step. This process can be done using either wet or semi-dry transfer methods. Wet transfer is often preferred for larger proteins, while semi-dry is quicker. Membrane types, such as nitrocellulose or PVDF, need to be selected based on specific requirements. After the transfer, it is essential to verify the successful transfer by staining the membrane with Ponceau S or similar dyes. Proper transfer efficiency ensures accurate quantification in the next stages.
Blocking and Antibody Incubation
Blocking the membrane is necessary to minimize non-specific binding. A blocking solution, like BSA or non-fat dry milk in PBS or TBS, is applied for a defined period. This step is crucial as it prevents background noise in the detection phase. After blocking, the membrane is incubated with specific primary antibodies against GFAP. The choice of antibody, including its dilution, should be optimized according to the sensitivity and specificity required. Incubation times vary, with overnight at 4°C being common.
Detection Methods
Detection methods are the final piece of the GFAP Western blotting methodology. Several approaches can be used, such as chemiluminescence, fluorescence, or colorimetric detection. The choice depends largely on the detection system in use. For chemiluminescence, substrates are added to the membrane, producing light that is captured using imaging systems. It is essential to calibrate the detection system to ensure consistent results. Accurate interpretation of results hinges on effective detection methods, highlighting the need for careful selection.
Summary: Proper methodology in GFAP Western blotting is vital for achieving reliable and reproducible results. Each step from sample preparation to detection must be executed with precision. This methodology serves as the foundation for advancing research in neurobiology.
Interpreting GFAP Western Blot Results
Interpreting the results from GFAP Western blot analysis is critical for understanding the role of GFAP in various neurobiological contexts. Accurate interpretation provides insights into astrocyte activation, responds to injury, and may offer diagnostic information. This section will discuss key aspects of interpretation, emphasizing how to accurately analyze band intensity, employ quantification techniques, and avoid common pitfalls.
Analyzing Band Intensity
Band intensity in a Western blot is often indicative of protein expression levels. In GFAP studies, stronger bands usually correlate with higher levels of the protein, while weaker bands suggest reduced expression. Here are important steps to consider:
- Control Samples: Always include control samples to benchmark band intensity. This establishes a baseline for comparison.
- Loading Controls: Utilize loading controls, such as beta-actin, to ensure equal amounts of total protein were applied to each lane. This helps normalize results.
- Visual Assessment: Carefully examine the degree of band intensity relative to controls. Visual discrepancies can offer preliminary insights but should be complemented by quantitative analysis.
Using imaging software can improve the accuracy of band intensity measurements. Proper calibration and consistent settings are essential to ensure reliability in results. Intensity analysis alone should not dictate interpretations; rather, it should work in tandem with other results.
Quantification Techniques
To derive meaningful insights from GFAP Western blots, quantification of band intensity is necessary. Here are key methods:
- Densitometry: This technique involves analyzing bands using software to measure optical density. The software provides numerical values representing the amount of GFAP protein present in the sample.
- Standard Curve: Preparing a standard curve with known concentrations of GFAP can help calculate protein levels in samples. This method increases accuracy by providing a reference for comparison.
- Relative Quantification: This approach compares the intensity of the GFAP band to a loading control band, expressing GFAP levels relative to that control. Such calculated values allow for normalization of variations in protein loading.
- Statistical Analysis: Employ statistical methods to assess the significance of changes in GFAP levels. This is fundamental in experimental studies where results may fluctuate due to biological variability.
Accurate quantification ensures that data drawn from GFAP Western blotting is robust and can be confidently interpreted for experimental objectives.
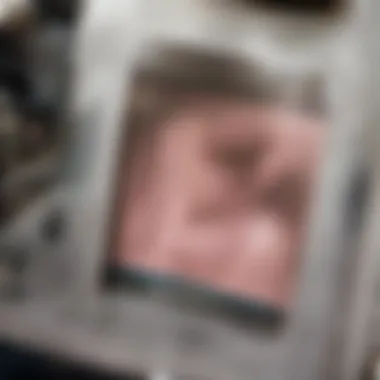
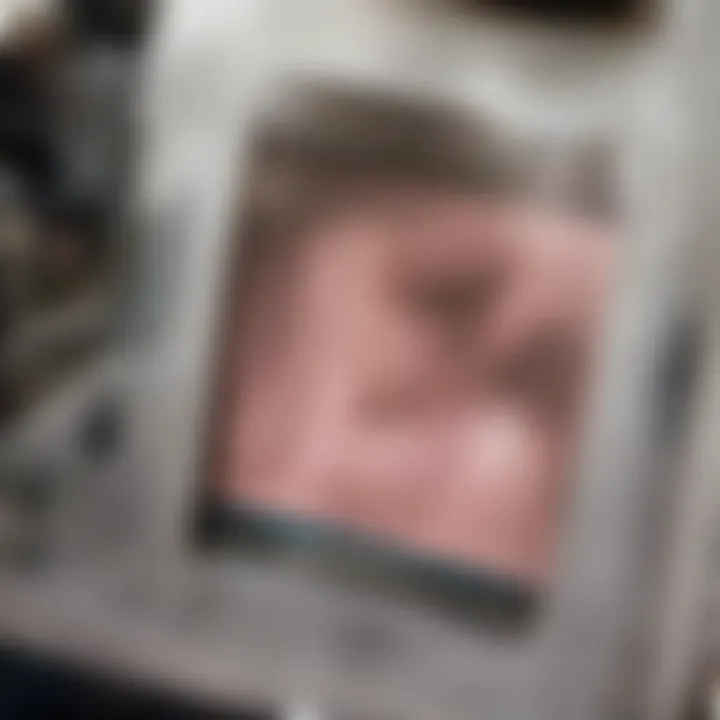
Common Pitfalls in Interpretation
Despite the utility of GFAP Western blotting, challenges exist in result interpretation. Understanding these pitfalls can enhance accuracy:
- Misinterpretation of Background Noise: Background signals can obscure results. Take care to differentiate specific signals from noise.
- Overlapping Bands: If GFAP bands overlap with bands from other proteins, this can complicate interpretation. Running additional controls or using different gel conditions may aid separation.
- Variability in Sample Sources: Different tissues or treatment conditions can cause variability in GFAP expression. Interpretation should consider the biological source of samples.
- Inconsistent Experimental Conditions: Minor variations in gel preparation, transfer techniques, or detection methods can affect results. Keeping constants in the experimental setup is essential for reliable outcomes.
Proper training in Western blot interpretation, combined with caution towards these pitfalls, ensures that findings contribute meaningfully to our understanding of GFAP's role in neurobiology.
Applications of GFAP Western Blot in Research
The application of GFAP Western blotting plays a crucial role in neurobiology research. Understanding GFAP's function and its relevance to various neurological conditions provides insights into fundamental brain mechanisms. This section explores three core areas where GFAP Western blotting is particularly impactful: studying neurodegenerative diseases, investigating brain injury, and examining allogenic conditions.
Studying Neurodegenerative Diseases
Neurodegenerative diseases such as Alzheimer's and Parkinson's diseases exhibit a complex interplay of pathological processes. GFAP, as a marker for astrocyte activation, serves as a significant indicator of these processes. In these conditions, GFAP levels are often found to be elevated, reflecting astrocyte response to neuronal damage. The ability to quantify GFAP through Western blotting allows researchers to correlate its expression with the disease stage, offering potential biomarkers for diagnosis and progression monitoring.
A few specific benefits of studying GFAP in neurodegenerative research include:
- Early Detection: Increased GFAP levels can signal early astrocytic response, aiding in diagnosis.
- Therapeutic Targets: Understanding GFAP dynamics can lead to the identification of novel therapeutic options.
- Pathophysiological Insights: GFAP levels provide a window into the neuroinflammatory processes central to these diseases.
Investigating Brain Injury
Brain injury, whether due to trauma, stroke, or other etiologies, initiates a cascade of cellular responses. Astrocytes play a pivotal role in this recovery process. GFAP Western blot analysis enables scientists to assess the extent of astrocytic activation following injury. An increase in GFAP can be observed post-injury, suggesting a reactive state that might impact neuronal recovery and repair.
Moreover, GFAP can be utilized to measure:
- Extent of Damage: Elevated GFAP levels can indicate the severity of injury.
- Recovery Process: Monitoring GFAP expression over time can give insights into healing trajectories.
- Inflammatory Response: The GFAP levels help assess the extent of neuroinflammation following brain injury.
Role in Allergenic Conditions
GFAP's relevance extends beyond neurodegenerative and injury contexts. Some studies indicate a connection between GFAP levels and allergic responses within the brain. Conditions such as allergic encephalomyelitis revealed changes in GFAP expression, suggesting a role in modulating inflammatory responses. GFAP detection through Western blotting offers researchers a pathway to explore how allergens may influence glial activity, thus impacting overall brain health.
Potential applications in allogenic contexts may include:
- Identifying Allergic Reactions: GFAP levels may serve as indicators of astrocytotic responses to allergens.
- Pathway Exploration: Understanding how allergens affect astrocyte function sheds light on the link between systemic conditions and neurological health.
The integration of GFAP Western blotting into research related to neurodegenerative diseases, brain injury, and allergic conditions showcases its versatility. Ongoing investigations will likely unveil further applications, solidifying GFAP's place in the cardiovascular neuroscientific community.
Technical Challenges in GFAP Detection
Understanding the technical challenges in GFAP detection is essential for researchers aiming to utilize Western blotting effectively. The analysis of glial fibrillary acidic protein (GFAP) levels can yield critical insights into various neurobiological conditions. However, several factors can interfere with the accuracy and reliability of results. Addressing these challenges not only improves the robustness of GFAP measurements but also enhances the interpretation of data in neurological research.
Sample Variability
Sample variability poses a significant challenge in GFAP Western blotting. Biological samples can differ greatly in composition due to various factors, including the source of the sample, handling methods, and even the condition of the subject from which the sample is drawn. This variability can lead to inconsistent GFAP expression levels, complicating data comparison across different experiments or studies.
To mitigate this, it is crucial to standardize sample collection and processing procedures. Using control samples can also help assess variability and improve reproducibility. Moreover, maintaining consistent conditions during experiments, such as temperature and buffer composition, is essential to minimize discrepancies in GFAP levels.
Antibody Specificity
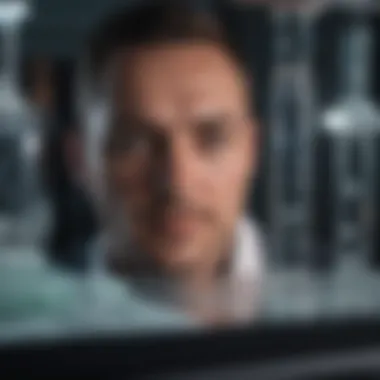
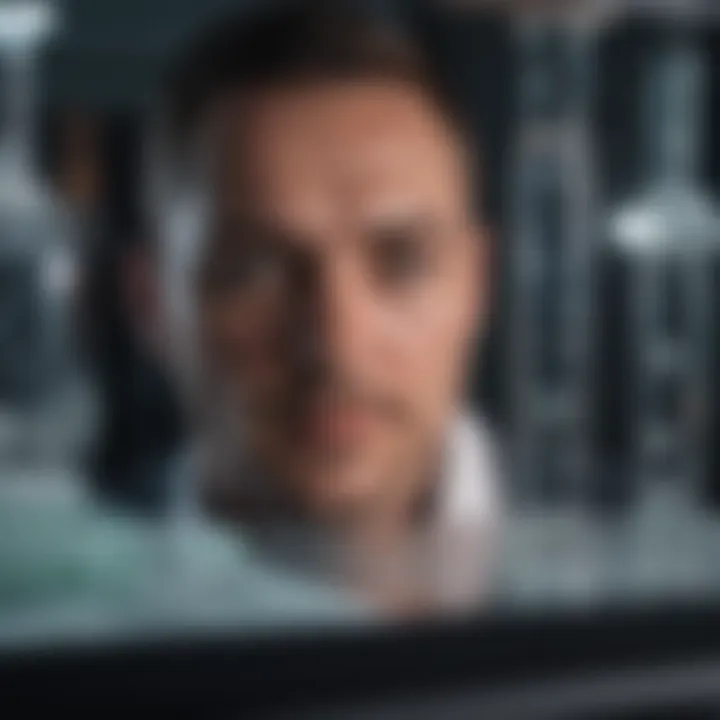
Antibody specificity is another critical element affecting GFAP detection. The choice of antibodies can greatly influence the reliability of Western blot results. Non-specific binding or cross-reactivity of antibodies can lead to background signals, which mask the true GFAP bands in the blot. This issue highlights the importance of validating antibodies before use in assays.
Careful selection of primary and secondary antibodies, based on their specificity to GFAP, is vital. Researchers should refer to manufacturer data and, when possible, conduct preliminary assays to test the suitability of antibodies in their experimental context. Utilizing methods like pre-absorption of antibodies can also reduce the chances of non-specific binding.
Optimizing Experimental Conditions
Optimizing experimental conditions is fundamental to achieving accurate GFAP measurements through Western blotting. Several parameters, such as gel concentration, electrophoresis time, and transfer methods, can significantly influence the outcome. Even variables like the dilution of antibodies and incubation times can alter the brightness and clarity of the GFAP bands.
One way to ensure consistency is to conduct a series of pilot experiments aimed at fine-tuning these conditions. For instance, adjusting the acrylamide concentration in the gel can provide better resolution for GFAP bands, particularly when analyzing samples with close molecular weights. Additionally, it is advisable to routinely assess the quality of the reagents used, as expired or improperly stored chemicals can lead to unpredictable results.
In summary, overcoming the technical challenges in GFAP detection is crucial for enhancing the reliability and validity of research findings. By addressing sample variability, ensuring antibody specificity, and optimizing experimental conditions, researchers can improve their understanding of GFAP's role in neurobiology.
"Attention to detail in these technical challenges not only fosters more reliable data but also promotes advancements in our understanding of neurological diseases."
Maintaining an informed approach to these barriers will ultimately contribute to better insights into astrocyte function and their implications in health and disease.
Future Directions in GFAP Research
Future directions in GFAP research promise to expand our understanding of glial fibrillary acidic protein beyond current paradigms. Researchers are keen to explore novel methods that enhance detection sensitivity and specificity. This is pivotal, as improved detection technologies can lead to better insights in neurobiology, especially concerning astrocyte function and pathology.
Advancements in Detection Technologies
Recent advancements in detection technologies are reshaping GFAP research. Techniques such as enhanced chemiluminescence or fluorescence-based detection systems offer improved sensitivity over traditional Western blotting methods. New antibody development, utilizing recombinant technologies, allows for higher specificity and reduced background noise. These advancements also promise faster processing times and lower sample volumes, which can be beneficial in clinical settings where sample availability is limited.
The integration of mass spectrometry with Western blotting is another significant advancement. This hybrid approach allows for not just the quantification of GFAP but also the assessment of post-translational modifications, providing a more comprehensive picture of its role in various conditions. Moreover, the emergence of automated platforms for Western blot analysis can decrease the variability often seen in manual techniques, ensuring reproducible results across different laboratories.
Integration of GFAP with Other Biomarkers
Integrating GFAP measurement with other biomarkers opens up new avenues for research. Biomarkers such as neurofilament light chain and S100B can provide complementary information about neuroinflammation and neurodegeneration.
By analyzing multiple biomarkers together, researchers can create more accurate models of brain pathology. This multifaceted approach could advance diagnostic methods, allowing for earlier detection of neurodegenerative diseases and monitoring of disease progression. Furthermore, it can assist in tailoring more effective therapeutic interventions based on a more holistic understanding of disease mechanisms.
Clinical Implications of GFAP Research
Clinical implications of GFAP research are vast and significant. As a biomarker, GFAP levels have the potential to provide critical insights into various neurological disorders. For instance, elevated GFAP is often observed in patients suffering from traumatic brain injury or neurodegenerative diseases.
Understanding GFAP dynamics could aid in risk stratification and management of patient care. Clinicians could utilize GFAP measurements to not only confirm diagnoses but also to monitor treatment responses. This could enhance personalized medicine approaches in neurology, ultimately leading to improved patient outcomes.
Moreover, the inclusion of GFAP levels in routine blood tests could shift diagnostic paradigms, making it easier to identify and treat neurological issues before they reach advanced stages. Therefore, prioritizing research in GFAP serves not just academic interests but directly impacts clinical practices and patient lives.
"Researching GFAP and its interactions with other biomarkers is a step towards revolutionizing diagnostics and interventions in neurology."
By focusing on these future directions, the scientific community may significantly enrich our comprehension of astrogliosis and its contributions to human health.
Epilogue
In summary, the relevance of GFAP in neurobiology and the application of the Western blot technique cannot be overstated. This article highlights how GFAP serves not only as a structural protein in astrocytes but also as a crucial biomarker in various neurobiological conditions. Understanding the intricacies of GFAP Western blotting is essential for researchers focused on dissecting the complexities of brain pathology.
One significant element discussed is the technique's methodology. It encompasses various stages, from sample preparation to detection methods. Each stage demands precision. This meticulous attention to detail aids in garnering reliable data, which is paramount in scientific research. The challenges, such as sample variability and antibody specificity, require researchers to continually optimize their protocols to ensure accuracy.
Furthermore, the potential clinical implications of GFAP research indicate a growing body of evidence linking GFAP levels to neurological diseases. Exploring these correlations is vital for developing targeted therapies and diagnostic tools that could improve patient outcomes.
The discussion of future directions also paves the way for advancements in the field. Integrating GFAP with other biomarkers could unlock new avenues for understanding neuronal injuries and diseases. As the science changes, so do the methodologies. Researchers must stay updated with technological advancements to leverage these tools effectively.
"A comprehensive grasp of GFAP Western blotting is instrumental for any neurobiologist aiming to contribute meaningfully to the field."
In reviewing the importance of GFAP as a biomarker and its role in research, it becomes clear that its study will remain a critical component in neurobiology. The conclusion not only wraps up the discussion but also emphasizes the need for ongoing research. Keeping GFAP applications in sight allows scientists to move forward effectively with innovative solutions to pressing questions in neurobiology.