Exploring Fibronectin Coating Techniques for Cell Cultures
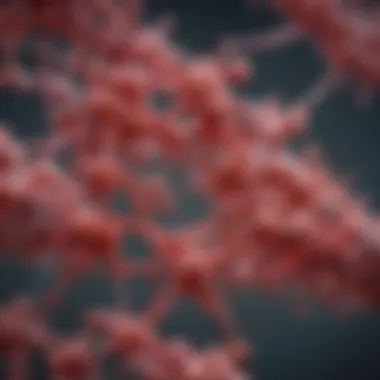
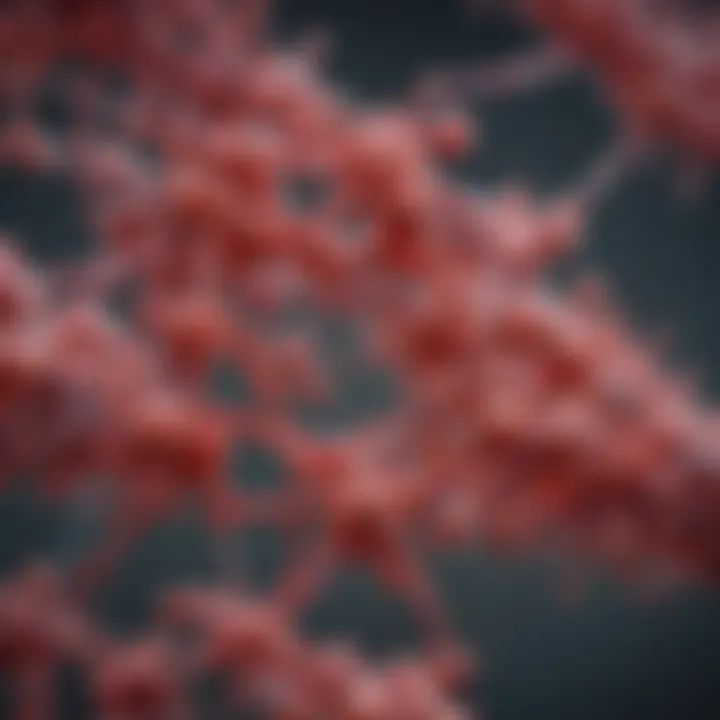
Intro
The development of reliable cell culture techniques is crucial in fields such as regenerative medicine, drug discovery, and tissue engineering. An often-overlooked aspect in optimizing these techniques is the importance of the extracellular matrix protein known as fibronectin. Coating plates with fibronectin has proven to be a significant game-changer in enhancing cellular adhesion and growth. This exploration takes a closer look at the multifaceted aspects of utilizing fibronectin as a coating agent, its properties, and its implications in various biomedical applications.
Intro to Fibronectin
Fibronectin plays a critical role in the field of cell biology and tissue engineering, making it a focal point for scholars, researchers, and industry professionals alike. As a multifunctional extracellular matrix (ECM) protein, its importance extends beyond mere structural support; it actively participates in various cellular processes. This understanding lays the groundwork for numerous applications, particularly in improving cell adhesion and proliferation.
Fibronectin's unique properties facilitate its use in various experimental setups, especially when coating laboratory plates. The plates become conducive to the growth and attachment of cells in a culture environment. This has significant implications for regenerative medicine and biomedical research.
Definition and Structure
Fibronectin is a glycoprotein crucial to cellular interactions. Structurally, it is composed of distinct structural domains that contribute to its versatility. The protein forms a dimer, meaning it’s made of two identical subunits joined by disulfide bonds. Its modular design allows fibronectin to engage with integrins on cell surfaces, serving as a pathway for signal transduction and cell-matrix attachments. The RGD (arginine-glycine-aspartic acid) sequence, prevalent in fibronectin, is particularly key to mediating cell adhesion.
Functional Roles in the Extracellular Matrix
Fibronectin serves various roles within the extracellular matrix. It provides a scaffolding for cells and is instrumental in organizational support within tissues. Its capacity to bind to other ECM components, like collagen and proteoglycans, underlines its role in maintaining tissue architecture. Fibronectin is also involved in wound healing, as it helps orchestrate the migration and proliferation of cells to the site of injury. The synthesis and degradation of fibronectin occur in a regulated manner, further emphasizing its importance in tissue homeostasis.
Importance in Cell Adhesion and Migration
The role of fibronectin in cell adhesion and migration is particularly noteworthy. By binding to integrins, fibronectin facilitates cells' attachment to the substrate, which is vital for their survival and functionality. Cell migration, required during processes like embryogenesis and immune responses, relies heavily on fibronectin's ability to guide cells through the ECM. This dynamic interaction not only affects how cells adhere but also influences their shape, movement, and orientation. Thus, understanding fibronectin can propel advancements in various biomedical applications, from drug delivery systems to tissue engineering.
Significance of Plate Coating
Coating plates with fibronectin is not just a procedural step; it forms the bedrock of numerous cellular processes. The significance of plate coating lies in its direct impact on various cellular functions essential for both basic and applied biological research. These coatings facilitate favorable environments that significantly enhance cell viability, growth, and behavior. Understanding this significance unveils the multifaceted roles that fibronectin plays in cell biology, emphasizing the need to optimize such coatings for specific applications.
Enhancing Cell Attachment
The act of cell attachment is crucial for cell survival and functionality. Fibronectin presents an ideal scaffold, offering specific binding sites that foster strong interactions between cells and the substrate. This process transforms the plates from mere surfaces into activated sites that promote stable adhesion.
When cells adhere effectively, they not only survive but thrive, enabling a rich and varied investigation into cellular responses under different experimental conditions. Enhanced attachment can be associated with increased levels of cytoskeletal organization and signaling pathways that are critical for subsequent cellular activities. Downstream effects can include upregulation of genes that promote growth and differentiation. Such changes are immensely beneficial for studies aiming to understand cellular mechanics and interactions.
Influencing Cellular Behavior
The manner in which cells behave is intricately tied to their environment, and here fibronectin plays a central role. By coating plates, fibronectin can dictate significant nuances in cellular behavior, including migration, proliferation, and differentiation. For example, when stem cells are cultured on fibronectin, they display increased motility and are more likely to commit to specific lineages compared to untreated plates.
Many of these behaviors are underpinned by various mechanical signals sent through the extracellular matrix (ECM), where fibronectin acts as a critical mediator. Moreover, altering the coating conditions can influence cell shape and size, further impacting gene expression and cellular fate. This emphasizes the versatility of fibronectin in scaffolding qualitative cellular behavior, laying the groundwork for innovative research applications.
Implications for Tissue Engineering
The fusion of fibronectin coatings with tissue engineering is an area that holds tremendous promise. As the field strives to develop biocompatible scaffolds for organ reconstruction or grafts, understanding how fibronectin influences cell behavior opens new routes for effective design. When conducive coatings are applied, cells exhibit not only enhanced attachment but also better versatility and durability, factors critical for developing successful tissue engineering strategies.
Furthermore, fibronectin’s role in guiding cell lineage specification is an area ripe for exploration. Using coated plates as a platform, researchers can further investigate how these properties contribute to tissue regeneration and repair.
"Fibronectin offers a dynamic interface that transforms basic research into practical solutions in regenerative medicine."
In summary, the significance of plate coating with fibronectin cannot be understated. It affects cellular attachment, influences behavior, and provides a critical foundation for applications in tissue engineering. The ongoing exploration of these areas is essential for advancing biomedical research and developing therapeutic strategies.
Methods of Coating Plates with Fibronectin
Coating plates with fibronectin is an essential technique that enhances cellular attachment and growth. This process is not just about coverage; it plays a significant role in creating an optimal environment for cellular activities. Understanding various methods to achieve these coatings can lead to improved reproducibility, efficiency, and effectiveness in biological experiments. Here, we will explore the most prominent methods used in the field: physical adsorption, chemical coupling techniques, and self-assembly techniques.
Physical Adsorption
Physical adsorption is often the simplest and quickest method for coating plates with fibronectin. This technique involves the passive attachment of fibronectin molecules onto the surface of the plate through van der Waals forces and hydrogen bonding. One of the main advantages of this approach is that it does not require any complex chemical reactions, making it user-friendly, particularly for beginners in the lab.
Key Benefits:
- Simple and Convenient: The process is straightforward, as it involves diluting fibronectin in a buffer solution and incubating it on the plate surface.
- Widely Applicable: This method can be utilized with various substrates, making it versatile across different lab settings.
- Retention of Biological Activity: Since it doesn't alter the fibronectin structure, the biological functionality is maintained, crucial for cell adhesion.
However, there are some considerations to keep in mind:
- Variability in Coating Thickness: The resulting thickness can vary, impacting the uniformity of cell behavior.
- Desorption Over Time: The stability of the coating might decline over time, especially under dynamic culture conditions.
Chemical Coupling Techniques
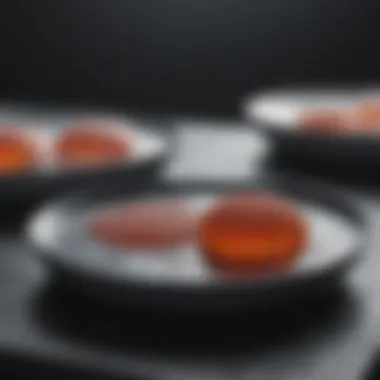
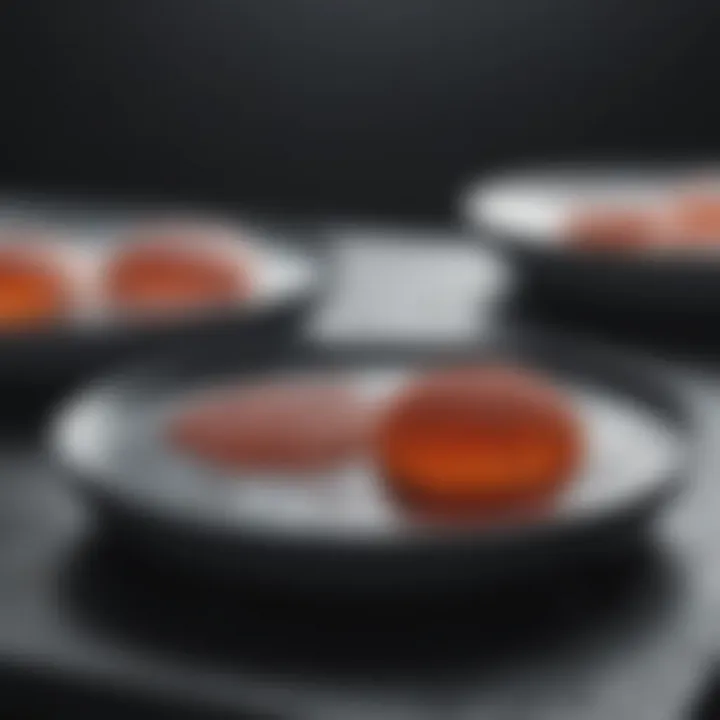
Unlike physical adsorption, chemical coupling techniques involve a more complex interaction where fibronectin is covalently bonded to the substrate. This technique often requires pre-treatment of the plate surface with activating agents that facilitate binding. The need for such treatments also means there's a steeper learning curve involved.
Key Benefits:
- Durable Coatings: The covalent bonds formed lead to stronger and more stable coatings that are less susceptible to desorption, especially in long-term cultures.
- Controlled Density: This method allows for more precise control over the density of fibronectin, enabling researchers to tailor the environment according to specific experimental needs.
- Enhanced Cell Response: Cells tend to respond better to covalently linked fibronectin due to more stable interactions, promoting increased adhesion and spreading.
Moreover, the following factors are essential:
- Surface Preparation: Proper surface modifications are critical and can require multiple steps, which may increase the time and complexity of the process.
- Potential Loss of Activity: If not performed carefully, there is a risk of altering fibronectin's biological activity during the bonding process.
Self-Assembly Techniques
Self-assembly techniques present a more innovative approach to fibronectin coating. These methods rely on the spontaneous organization of fibronectin molecules into a structured layer upon exposure to a suitable environment. This could include changes in temperature or ionic concentration to promote the self-organization of proteins into nanostructured layers.
Key Benefits:
- Natural Orderliness: Self-assembled layers can mimic more closely the natural extracellular matrix's structure, potentially improving cell behavior and functionality.
- Scalability: These methods can be scaled up for industrial applications with relative ease, allowing for large production of coated plates.
- Versatility with Other ECM Components: Self-assembly can often be achieved alongside other extracellular matrix proteins, allowing for composite coatings that enhance functionality.
However, challenges exist:
- Complexity of Process: The methods can be quite intricate and may require precise control over conditions to ensure effective assembly.
- Reproducibility Issues: Variability in conditions can lead to differences in coating quality, which needs careful monitoring.
In summary, the choice of coating method is pivotal in fibronectin research. Factors such as desired stability, biological activity, and specificity all play a role in selecting the most appropriate coating technique.
"Selecting the right coating technique is crucial not just for experimental fidelity but also for enhancing the potential applications in regenerative medicine and cellular therapies."
This comprehensive examination of coating methods emphasizes how technical choices will ultimately influence cellular interactions and research outcomes. As the field evolves, optimizing these methods will pave the way for more effective applications and a deeper understanding of fibronectin's role within the extracellular matrix.
Optimal Conditions for Coating
The performance and effectiveness of fibronectin-coated plates depend significantly on optimizing several conditions during the coating process. Establishing the right parameters not only ensures the stability of the fibronectin layer but also maximizes its functionality, which is critical for applications involving cell attachment and growth. This section emphasizes key elements such as temperature, pH, concentration, coating time, and how to evaluate the quality of the applied coating.
Temperature and pH Considerations
When coating plates with fibronectin, both temperature and pH levels are fundamental considerations. The fibronectin protein is sensitive to thermal and chemical variations, which can affect its structural integrity and functional performance. Generally, a temperature range between 4°C and room temperature (20-25°C) is recommended for most coating procedures. Lower temperatures can slow the denaturation process of proteins but may also influence the adsorption kinetics on surfaces.
Moreover, the pH of the coating solution plays a crucial role in maintaining the protein's biological activity. A neutral pH (around 7.4) is often ideal, as it helps preserve fibronectin's conformation and functionality. Deviating from this pH can lead to changes that may adversely affect cell adhesion. Notably, excellent adhesion is observed when the coating solution mimics physiological conditions. Thus, maintaining the right balance in temperature and pH becomes crucial for favorable binding interactions at the cellular level.
Concentration and Coating Time
The concentration of fibronectin in the coating solution directly impacts the quality of the coating. For most applications, concentrations ranging from 1 to 10 µg/mL are commonly recommended. If the concentration is too low, the coating may be insufficient for effective cell adhesion. Conversely, excessively high concentrations can lead to thick layers that may mask critical binding sites on the surface, thus hindering cell interactions. Therefore, finding that sweet spot is essential; experimenters often start at a mid-range concentration and adjust based on observed outcomes.
Additionally, the time allotted for coating can affect the surface's properties. The typical coating time can vary from one hour to overnight, depending on the chosen method and specific conditions. For example, prolonged exposure can improve the density of the fibronectin layer but might also increase the likelihood of degradation. A careful balance allows researchers to tune these conditions based on the specific requirements of their experiments.
Evaluation of Coating Quality
Once plates are coated, it’s vital to evaluate the quality of the fibronectin layer. An adequate assessment ensures that the coating meets the necessary standards for subsequent applications. Several methods can be employed to evaluate the coating quality:
- Attenuated Total Reflectance Fourier Transform Infrared (ATR-FTIR) Spectroscopy: This technique allows for the characterization of molecular interactions between fibronectin and the substrate.
- X-ray Photoelectron Spectroscopy (XPS): It provides detailed elemental analysis and can be used to determine the functional groups on the surface after coating.
- Cell Adhesion Assays: These assays quantitatively measure how well cells adhere and proliferate on the fibronectin-coated surfaces, giving insights into the functional efficacy of the coating.
- Biochemical Quantification: Enzyme-linked immunosorbent assays (ELISA) can ascertain the amount of residual fibronectin on the surface post-coating.
Ultimately, a comprehensive evaluation of the coating quality is not just advantageous; it is essential in ensuring that the fibronectin facilitates desired cellular interactions and supports critical research or clinical applications. As researchers dive deeper into their experiments, the significance of these optimal conditions becomes increasingly clear, paving the way for innovative breakthroughs in cell biology and regenerative medicine.
Comparative Analysis: Fibronectin vs. Other ECM Proteins
Comparing fibronectin with other extracellular matrix (ECM) proteins provides crucial insight into its unique properties and applications, especially in the realm of cell culture and regenerative medicine. Fibronectin, due to its versatile nature, plays a pivotal role in cellular activities such as adhesion, growth, migration, and differentiation. By evaluating how fibronectin stacks up against other key proteins like collagen and laminin, researchers and professionals can better discern the optimal conditions for enhancing cell functions in laboratory and clinical settings.
Collagen
Collagen is often regarded as the primary structural protein found in many organisms, making up a significant portion of the extracellular matrix in a variety of tissues. It provides tensile strength and structural support, which are paramount for tissue integrity. However, while collagen is exceptional in providing mechanical stability, it does not facilitate cellular interactions as effectively as fibronectin.
- Cellular Interactions: Fibronectin possesses specific cell-binding domains that promote cellular adhesion, thus influencing cell behavior more dynamically than collagen.
- Dynamic Functionality: Unlike collagen, fibronectin can undergo conformational changes that enhance its ability to interact with various integrins and other ECM components, which is critical for processes like wound healing and tissue repair.
- Role in Development: In embryonic development, fibronectin is vital for cell migration and tissue organization, while collagen is primarily responsible for establishing physical structures post-development.
In summary, while collagen provides foundational support, fibronectin offers dynamic cellular engagement, illustrating the complementary roles these proteins play in the ECM.
Laminin
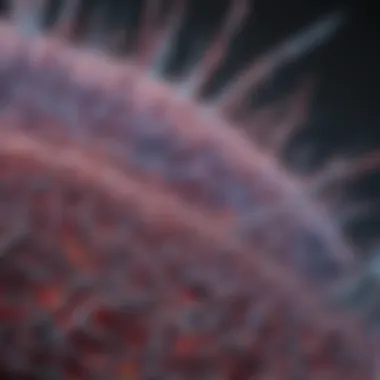
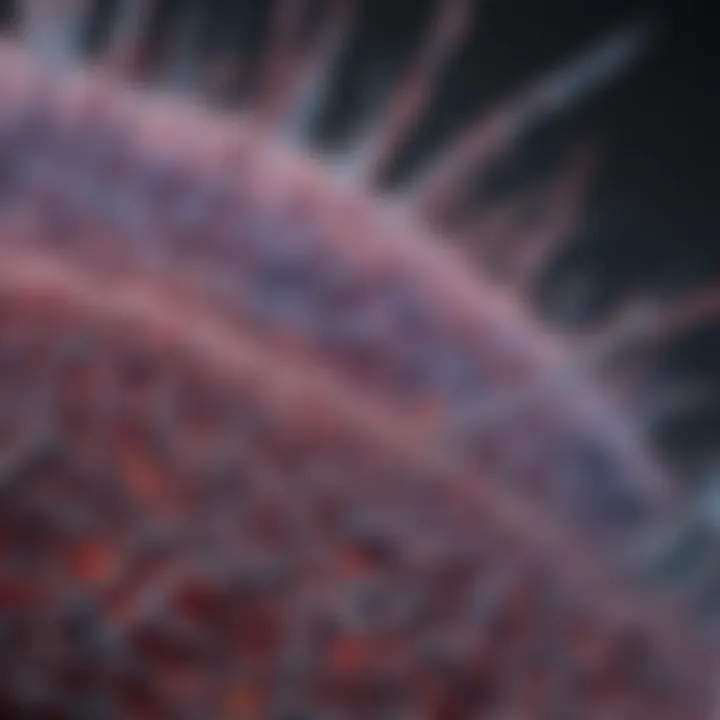
Laminin is another essential component of the extracellular matrix, well-known for its role in influencing cell adhesion, differentiation, and migration. It is typically found in the basal lamina, a specialized layer of the ECM. Compared to fibronectin, laminin also presents certain advantages and distinct functions:
- Structure and Function: Laminin's structure includes three polypeptide chains that form a cross-shaped molecule, which is particularly effective in binding cells through specific receptors, enhancing cell attachment and signaling.
- Cell Type Specificity: Laminins are highly specific to certain cell types and tissue environments, making them critical for specialized functions, such as in the nervous system and muscle tissue. However, fibronectin can generalize its role across multiple cell types, providing broader applicability in various research scenarios.
- Interplay with Fibronectin: Interestingly, fibronectin and laminin can work in concert within the ECM, where fibronectin can enhance the attachment of cells to laminin, thus optimizing the cell adhesion process.
The choice between using fibronectin or laminin often depends on the specific requirements of a given experiment or therapy, highlighting their unique yet complementary properties.
Other ECM Components
Beyond collagen and laminin, the extracellular matrix comprises various proteins such as proteoglycans, elastin, and fibulin, each with distinct functionalities:
- Proteoglycans: These molecules provide hydration and resistance to compressive forces, crucial for maintaining the structure of cartilage. However, they do not actively promote cell adhesion, a key function of fibronectin.
- Elastin: Known for its elasticity, elastin plays a vital role in tissues requiring flexibility. While it provides structural support, it lacks the cell-adhesive properties that fibronectin showcases.
- Fibulin: This provides structural support within tissues, aiding in ECM assembly. However, it does not have the adhesive traits specific to fibronectin.
Application in Stem Cell Research
The exploration of fibronectin's role in stem cell research reveals how vital this protein is in optimizing cell culture conditions. Fibronectin coatings on plates create a microenvironment that enhances cell adhesion, promoting optimal conditions for stem cells. This is significant because stem cells require specific physical and molecular cues to maintain their unique characteristics. Given that stem cell research holds enormous potential for regenerative medicine, understanding how fibronectin influences these cells can be a game-changer in scientific and clinical applications.
Role in Stem Cell Maintenance and Differentiation
Fibronectin plays a crucial role in stem cell maintenance by influencing cell behavior through signal transduction pathways. When stem cells interact with fibronectin, it triggers various cellular mechanisms that support their undifferentiated state. This interaction is not just about sticking to a surface; it's about creating a favorable environment where signals are exchanged between the stem cells and the extracellular matrix. Research demonstrates that plated stem cells on fibronectin-coated surfaces show increased expression of pluripotency markers.
Factors such as the thickness of the fibronectin layer and the concentration used can alter these outcomes, highlighting the need for careful protocol optimization. With the right coating conditions, researchers can significantly improve the yield of undifferentiated stem cells, which is particularly essential for applications in gene editing and cell therapy.
Influence on Pluripotency
Pluripotency, or the ability of stem cells to differentiate into any cell type, is influenced by their interaction with fibronectin. When stem cells are cultured on fibronectin surfaces, they exhibit a tendency to remain in a pluripotent state. This occurs because fibronectin contributes to the expression of genes that maintain pluripotency. Through various studies, it's become clear that the integration of fibronectin can modulate the balance between self-renewal and differentiation, which is fundamental for advancing therapy applications.
The influence of fibronectin does not act in isolation; it interacts with various signaling pathways. For example, the Integrin signaling pathway, heavily involved in cell-extracellular matrix communication, becomes active upon fibronectin engagement. This combined action helps stabilize the stem cell phenotype, which researchers are keen on harnessing to improve regenerative techniques in medicine.
Case Studies Highlighting Success
There have been multiple case studies that underscore the successful application of fibronectin in stem cell research. One notable example is a study conducted at Stanford University that explored the impact of fibronectin surface coatings on human embryonic stem cells. The results indicated an increased proliferation rate and enhanced viability of the stem cells when cultured on fibronectin-coated plates compared to standard conditions.
Another pertinent case involved researchers at the Massachusetts Institute of Technology, who utilized fibronectin to facilitate the differentiation of induced pluripotent stem cells (iPSCs) into neural progenitor cells. Their findings demonstrated that fibronectin not only supported efficient adhesion but also improved the differentiation efficiency, presenting a promising avenue for neural tissue engineering.
"The integration of fibronectin into stem cell culture protocols presents transformative opportunities, driving advancements in regenerative medicine that previously seemed unreachable."
In summary, fibronectin's application in stem cell research does not merely enhance cell culture conditions; it opens up new horizons for therapeutic interventions leveraging the complex biology of stem cells. As researchers continue to unravel the intricacies of these interactions, the role of fibronectin is poised to be a cornerstone in the future of biomedical applications.
Integrating Fibronectin into Bioreactor Systems
In the realm of tissue engineering and regenerative medicine, bioreactors play a pivotal role in providing controlled environments for cell growth and differentiation. Integrating fibronectin into bioreactor systems opens a new chapter in optimizing these environments, specifically designed to boost cell activity. Given its well-documented significance in cellular adhesion and migration, incorporating fibronectin in these systems is not just beneficial but crucial.
Fibronectin enhances the physical properties of the culture substratum, making it more conducive to cell attachment. This can be particularly important for cells grown in suspension, where adhesion to a solid surface is vital for growth. The sheer versatility of fibronectin in the bioreactor setup underscores its importance and its synergistic role in supporting cellular functions while mitigating challenges presented by suspension cultures.
Enhancing Cell Growth in Suspensions
For cells cultured in suspension, achieving optimal growth can be daunting. The lack of a stable surface often results in reduced proliferation rates and can complicate harvesting. However, when fibronectin is utilized, it provides an adhesive framework that encourages cells to attach, even in a fluid environment. This adhesion is key, as it allows the cells to receive the requisite signals from their surroundings for growth and division.
Utilizing fibronectin in suspension cultures can lead to several advantages, such as:
- Improved Viability: Cells, particularly stem cells, benefit from enhanced survival rates when they can adhere and spread, reducing stress and apoptosis.
- Increased Proliferation: Attachment fosters a more favorable microenvironment, essential for rapid cell division.
- Higher Yield: With better growth conditions, researchers can achieve more significant quantities of cells, which is paramount in clinical applications.
Incorporating fibronectin modifies the rheological properties of the culture medium, enhancing not just cell adherence but also promoting cell signaling pathways that are crucial for growth and maintenance.
Applications in Regenerative Medicine
The application of fibronectin in bioreactor systems extends far beyond mere cell growth. It plays a role that could arguably revolutionize aspects of regenerative medicine. As researchers explore tissue engineering solutions, they find that fibronectin not only offers structural support but also influences cellular differentiation.
Some notable applications include:
- Tissue Repair and Regeneration: Fibronectin can guide the formation of new tissues in damaged areas, providing an essential scaffold for cells.
- Cellular Therapy: Dependencies on stem cells in therapies promote an environment fostering their survival and differentiation, critical for effective treatments.
- Biomimetic Constructs: Creating three-dimensional tissue models is possible by using fibronectin to inspire cell behavior, enabling scientists to design more effective therapies for a variety of diseases.
"The integration of fibronectin into bioreactor systems is not just a technical enhancement; it represents a significant advance towards more effective regenerative treatments."
Conclusively, the integration of fibronectin into bioreactor systems propels the field of regenerative medicine into new territories, enabling improved growth conditions while paving the way for innovative therapeutic advancements. As researchers continue to navigate the complexities of human biology, the potential of fibronectin remains a promising beacon in bridging the gap between cellular biology and clinical application.
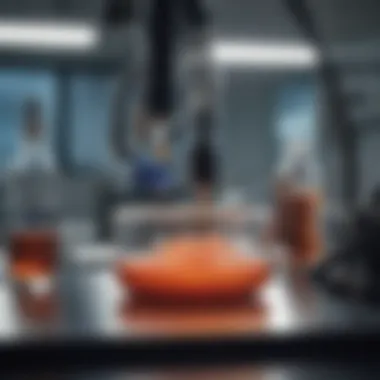
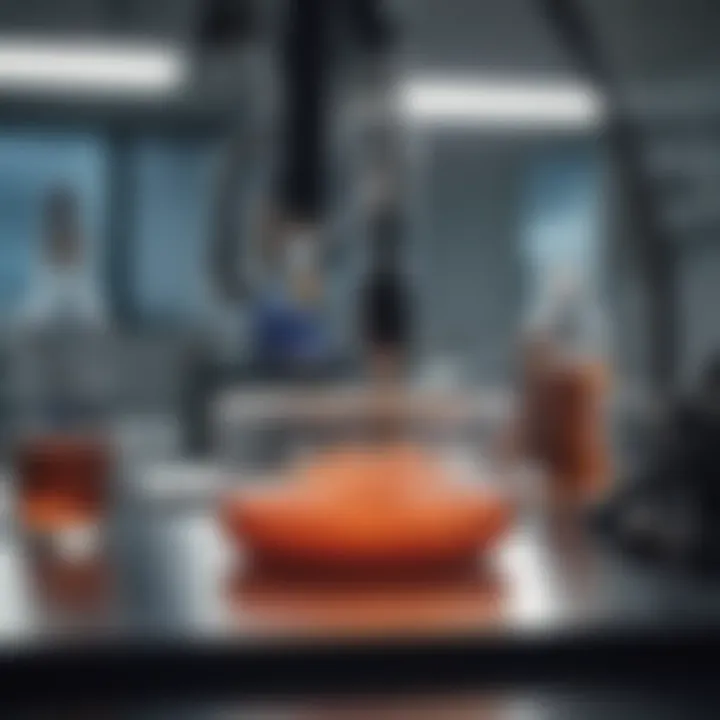
Challenges in Fibronectin Coating
When discussing the application of fibronectin in cell culture, it's essential to address the challenges associated with its coating on plates. Understanding these challenges can better inform researchers and professionals about potential pitfalls and how to navigate them effectively. Variability in coating protocols and stability concerns are two of the primary issues that might arise when employing fibronectin.
Variability in Coating Protocols
One of the prominent challenges that comes along with fibronectin coating is the variability in the protocols used. Each laboratory may adopt different methods, concentration levels, or even temperature settings when handling fibronectin, which can yield drastically different results.
This variability can lead to inconsistent cell attachment and growth across experimental setups. For instance, if one lab applies a 5 µg/ml concentration while another opts for 10 µg/ml, the outcomes in terms of cell proliferation could vary significantly. Even the most minor tweak in the environmental conditions, such as humidity or exposure to light, may impede uniformity.
To enhance reliability, it might be advantageous for researchers to standardize their protocols. This would involve a thorough documentation of each experimental condition, enabling reproducibility across studies. Moreover, conducting a comparative analysis of different protocols could help isolate optimal conditions that lead to better outcomes in cell culture.
Stability and Degradation Concerns
Stability is another pressing concern when coating plates with fibronectin. The biological activity of fibronectin can diminish over time due to degradation, particularly if exposed to unfavorable environmental conditions. Factors such as temperature fluctuation, pH levels, and even the presence of proteolytic enzymes can hasten this degradation.
To mitigate these challenges, it's crucial to consider storage and handling practices. For example, fibronectin should be stored at low temperatures and protected from light to preserve its activity. During its application as a coating, any prolonged exposure to ambient conditions should be avoided as much as possible. Additionally, ensuring that the pH levels remain consistent can help maintain fibronectin's structural integrity.
In summary, tackling the challenges of fibronectin coating involves understanding the variability in protocols and ensuring stability through careful handling and storage practices. It's vital to approach this process with diligence and standardization to reap the benefits of enhanced cell adhesion and proliferation that fibronectin can offer.
Key Takeaway: Variability in coating techniques and degradation risks necessitate standardized protocols for optimal use of fibronectin in cell culture applications.
Recent Advances in Fibronectin Research
Recent developments in fibronectin research have opened new avenues for improving cell culture techniques and biomedical applications. Understanding these advances is crucial for grasping how fibronectin’s fundamental properties can be leveraged in both laboratory and clinical settings. Researchers have been particularly focused on enhancing the techniques and materials used in coating plates with fibronectin, leading to more reliable and effective outcomes in various experiments.
These innovative techniques often aim to increase the binding efficacy of fibronectin while also addressing challenges related to stability and degradation. A deeper exploration of this topic will reveal not only the methods that have emerged but also their tangible benefits for cell-based research and applications.
Innovative Coating Techniques
Recent years have witnessed a surge in pioneering coating techniques for applying fibronectin to plates. These approaches include several promising methods that have shown improved outcomes compared to traditional techniques.
- Electrospinning: This technique utilizes an electric field to produce fibers from polymer solutions containing fibronectin. The resulting mat can mimic the natural extracellular matrix, allowing cells to adhere more acutely and establish a better environment for growth.
- 3D Printing: 3D printing technology has started to play a significant role in fibronectin coating. By customizing scaffold structures at the micro-level, researchers can layer fibronectin effectively to enhance the interaction between the cells and the coated surface.
- Micro-patterning: This method is used to control the spatial arrangement of fibronectin on the plate surface. By designing specific patterns, researchers can direct cell attachment and function more predictably, enhancing cellular behavior in desired manners.
These techniques not only cater to varied experimental designs but also highlight a move towards precision in cell culture applications with fibronectin.
Impact of Nanotechnology
Nanotechnology has significantly transformed the realm of fibronectin research, focusing on harnessing the unique properties of nanoscale materials. The integration of nanotechnology in fibronectin coating has several implications that could shape the future of cell biology and regenerative medicine.
- Improved Adhesion: By utilizing nanoparticles, researchers can enhance the adhesive properties of fibronectin. This results in stronger cell attachment and potentially improved viability in cultures.
- Targeted Delivery: Nanotechnology allows for the targeted delivery of fibronectin-coated nanoparticles to specific cellular environments. This precision could improve the therapeutic effectiveness in regenerative medicine.
- Enhanced Surface Properties: Nanostructuring the fibronectin layer can alter its surface properties, leading to improved interactions between fibronectin and cells, which can ultimately lead to better cell signaling and functionality.
"The incorporation of nanotechnology into fibronectin research has the potential to revolutionize approaches in tissue engineering and regenerative medicine, making it a focal point of contemporary studies."
Overall, advancements in coating techniques and the application of nanotechnology present significant opportunities for enhancing the effectiveness of fibronectin in various biological applications, positioning this area of research as vital for ongoing scientific pursuits.
End and Future Directions
In light of the comprehensive exploration presented in this article, it is clear that the role of fibronectin in cell culture environments goes beyond mere coating. As we’ve delved into its physiological significance, methods of application, and the challenges encountered, the need for continued research and development cannot be overstated. The importance of selecting the right techniques and optimizing conditions for effective fibronectin coating is pivotal. This often dictates not just cell adhesion, but also their growth and differentiation.
Advancements in technology reinforce the ability to enhance fibronectin's efficacy. For instance, integrating innovative coating techniques can pave the way toward improved biological responses from cells. Thus, one may ponder how these developments may bear fruit in clinical applications.
Looking ahead, the exploration of fibronectin’s utility in regenerative medicine appears promising. Conditions such as diabetes and cardiac issues might find new hope in therapies leveraging proficient cell scaffolding. In addition, there's a growing interest in combining fibronectin with other biomaterials that could lead to synergistic effects and bespoke solutions for tissue engineering. As scientists and healthcare professionals pursue a deeper understanding, the window for clinical implementation continues to widen, fostered by interdisciplinary collaborations and breakthroughs in materials science.
"Continuous exploration and fine-tuning of fibronectin applications may redefine the landscape of cellular therapy and tissue regeneration."
Summary of Key Insights
The investigation into fibronectin coating has revealed significant insights that elevate its status in biological research. First off, fibronectin serves as an essential facilitator for cell adhesion, impacting cellular behavior profoundly. This is critical for researchers aiming to create environments that allow optimal cellular function and growth. Moreover, understanding the various coating methods, from physical adsorption to self-assembly, equips scientists with tools to tailor conditions based on specific experimental needs.
In summary:
- Contribution to Cell Adhesion: Fibronectin is crucial for establishing an effective interface between cells and substrates.
- Diverse Coating Techniques: Multiple methods exist, each with unique advantages that can be exploited depending on the application.
- Impact on Development: Its role extends into guiding cell fate and behavior, crucial for advancements in regenerative medicine.
Potential for Clinical Applications
The clinical applications of fibronectin coating are vast and varied, presenting a horizon filled with potential. As healthcare advances merge with biotechnological developments, fibronectin's ability to enhance the survival and function of cells opens new avenues for treatment methodologies.
Potential avenues include:
- Tissue Engineering: Coating matrices in tissue scaffolds with fibronectin holds promise for rebuilding damaged or diseased tissues.
- Stem Cell Therapy: By optimizing conditions for stem cell cultures, clinicians might improve the efficiency of stem cell-derived therapies.
- Targeted Drug Delivery: Fibronectin could be used for creating targeted delivery systems, improving therapeutic precision while minimizing side effects.
As the field progresses, documentation of successful clinical translations will be crucial in solidifying fibronectin's role in next-gen therapies. Through continuous research efforts and clinical evaluations, the prospect of employing fibronectin in patient care becomes not just a possibility, but a promising reality.