Exploring MACS: Techniques and Applications in Cell Biology
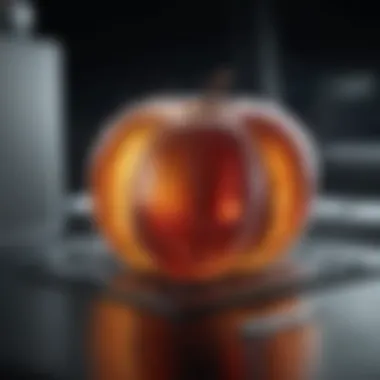
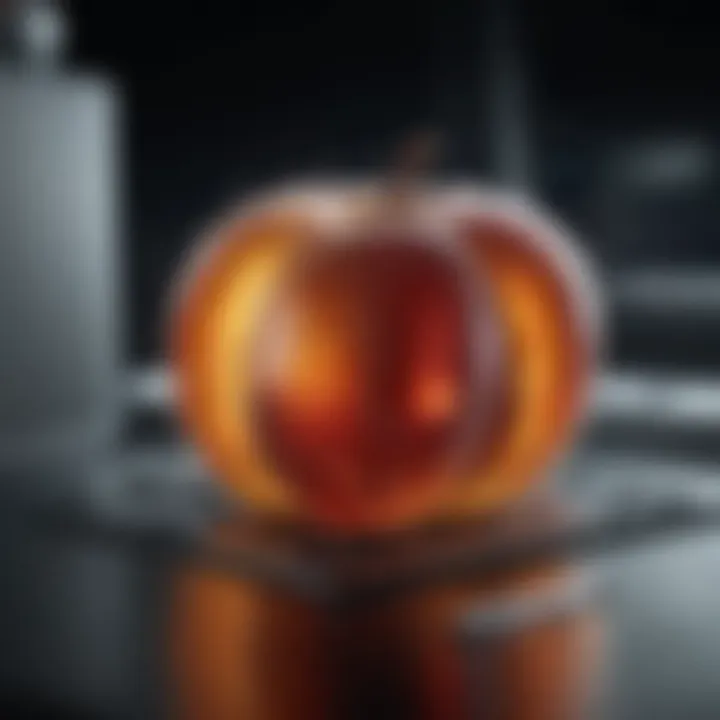
Intro
Magnetic Activated Cell Sorting, often abbreviated as MACS, is a powerful methodology that has revolutionized how scientists approach the study and manipulation of cells. The technique relies on magnetic fields to facilitate the separation of target cells from a diverse mixture, enabling researchers to hone in on specific populations that may otherwise be obscured by the vast majority of other cell types. Through this article, we will unpack the fundamental principles underpinning MACS and delve into various applications across disciplines such as immunology and regenerative medicine.
The potential of MACS to isolate certain cell types with such precision makes it an invaluable tool. Be it for studying immune responses or for therapies that hinge on stem cell engineering, understanding the ins and outs of MACS can lead researchers down avenues of innovation that were previously thought infeasible. As we explore the mechanisms of MACS and assess its wider implications, this narrative aims to bring clarity to both seasoned experts and newcomers in the field.
Prolusion to MACS Technology
The landscape of cellular biology has been forever altered with the advent of Magnetic Activated Cell Sorting (MACS). This technique, pivotal for cell separation, enables researchers to isolate specific cell populations from a complex mixture. The proficient use of MACS can significantly enhance both basic research and clinical applications. This section will dive into the historical backdrop and definition, as well as shed light on its importance.
Historical Background of MACS
The notion of separating cells based on their physical or biochemical characteristics is not new. Historically, the roots of cell sorting can be traced back several decades, evolving alongside advancements in molecular biology. The catalyst for MACS can be found in the exploration of magnetic beads. These beads, developed in the late 1970s, were initially used in various assays and diagnoses. By the 1990s, the concept took on a life of its own. Scientists recognized that they could coat magnetic beads with unique antibodies to selectively bind to target cells. This shift in thought translated into the practical application of MACS.
As researchers sought more efficient ways to analyze cells, MACS emerged as a response to the limitations of conventional techniques like fluorescence-activated cell sorting (FACS). The historical development of MACS represents an intersection of engineering and biology, creating a system where targeted selection is not just a dream but a tangible reality.
Defining MACS
So, what exactly is MACS? At its core, Magnetic Activated Cell Sorting stands as a technique employed to segregate cells based on magnetic properties. It operates on a simple yet effective principle: when a mixture containing diverse cell types is exposed to a magnetic field, only the cells tagged with magnetic beads (which are bound to specific cell surface markers) will be drawn towards the magnet.
There are a few key points to highlight:
- Magnetic Beads: These are often coated with antibodies that specifically attach to the cells of interest. When introduced to the cell mixture, these beads function like labels, enabling the retrieval of the desired cells.
- Cell Selection: The beauty of MACS is rooted in its specificity. It allows for precise targeting, meaning researchers can isolate, say, CD4+ T cells from a blood sample, all while leaving the rest untouched.
- Applications: From cancer research to stem cell studies, MACS has demonstrated its versatility and significance across multiple disciplines.
Fundamental Principles of Magnetic Activated Cell Sorting
Magnetic Activated Cell Sorting, or MACS, represents a pivotal innovation in biomedical research and clinical applications. This technology hinges on several fundamental principles, primarily focusing on the intricacies of magnetic bead technology, cell labeling mechanisms, and the physics of magnetic separation.
Understanding these principles is crucial for recognizing how MACS enhances cell sorting efficacy and specificity. As researchers delve into the complexities of cell populations, a clear grasp of MACS principles offers insights not only into its operational success but also into its potential to affect various fields from immunology to personalized medicine.
Magnetic Bead Technology
MAGNETIC BEAD technology is at the core of MACS. The concept involves tiny beads, often made of a polymer, which are coated with antibodies that specifically bind to the target cells of interest. Once these beads are mixed with a cell suspension, they attach themselves to the desired cells through specific antigen-antibody interactions.
- Types of Beads: These beads can vary in size and magnetic properties, influencing their effectiveness in cell sorting tasks. Generally, superparamagnetic beads are favored for their high sensitivity.
- Surface Chemistry: The choice of antibodies used to coat these beads is critical. The right pairing ensures that the beads can efficiently latch onto the target cells, allowing for successful sorting.
This technology not only augments sorting precision but also ensures that the cells retain their viability post-separation. Without magnetic beads, traditional methods often faced challenges in dealing effectively with heterogeneous cell mixtures.
Cell Labeling Mechanisms
Cell labeling mechanisms are pivotal as they dictate how well the magnetic beads can identify and bind to the target cell populations. There are two primary methods for labeling cells: direct labeling and indirect labeling.
- Direct Labeling: This approach entails the direct attachment of magnetic beads to the target cells. This method is generally simpler and results in a more straightforward sorting process. However, it may influence the cell's functionality due to the added mass of the beads.
- Indirect Labeling: This method employs secondary antibodies that bind to the primary antibodies already attached to the target cells. While this method can enhance specificity, it is often more complex and time-consuming.
Both labeling mechanisms guide researchers in effectively isolating desired cells, which can subsequently be analyzed for further research or therapeutic applications. By understanding the labeling processes, practitioners can select the most appropriate strategy based on their specific requirements.
Magnetics in Cell Separation
The physical principles underpinning the separation of labeled cells from unlabeled ones rely on the application of a magnetic field. This is a critical juncture in the MACS process. Once the cells are labeled with magnetic beads, they are subjected to a magnetic field that causes the bead-bound cells to move towards the magnet, while the unlabeled cells remain in the suspension.
- Gradient Fields: The power of MACS comes from utilizing magnetic gradient fields, which selectively control the movement of cells based on their labeling, resulting in highly purified cell populations.
- Continuous Flow Systems: This allows for the rapid processing of large volumes of cell suspensions.
MAGNETIC field manipulation allows for the efficient partitioning of mixed cells, making MACS a compelling choice over conventional cell separation methods. The physics of magnetics couples with biochemical interactions, creating a harmonious balance that facilitates advanced sorting capabilities.
"The strength of MACS lies in its combined focus on magnetic technology and biological specificity; it’s a game changer in the field of cell biology."
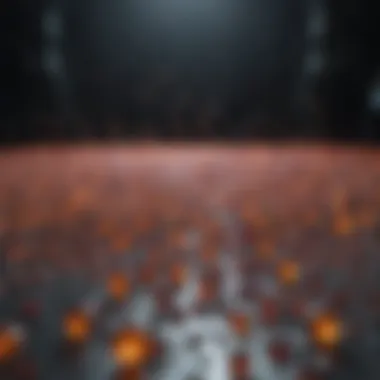
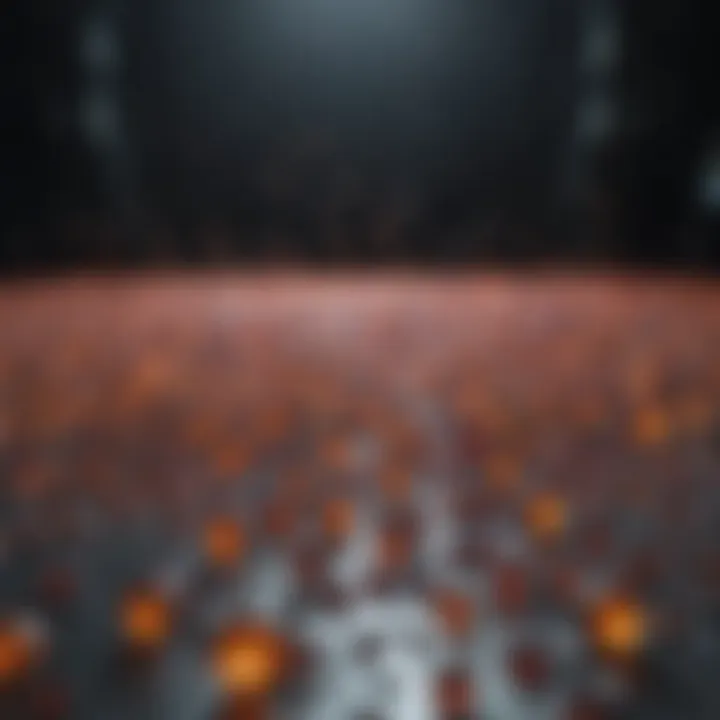
In closing, the fundamental principles of MACS provide the underpinnings essential for its success. With advancements in magnetic bead technology, sophisticated labeling techniques, and a solid understanding of magnetic separation dynamics, researchers and clinicians alike are better equipped to navigate the complexities of cellular analysis. This technology not only enhances the quality of research outcomes but also bridges the gap between laboratory findings and clinical applications.
Operational Methodologies of MACS
The operational methodologies of Magnetic Activated Cell Sorting (MACS) are crucial to understand because they outline the actual processes involved in leveraging this advanced technology for effective cell separation. This section dives into how preparations, applications of magnetic forces, and recovery techniques form the core of MACS, underscoring their significance in both research and practical applications. Effective methodology not only enhances the operational efficiency of MACS but also ensures the integrity and viability of the sorted cells, making the entire process reliable for various scientific endeavors.
Preparation of Cell Samples
Before the MACS process can begin, preparing cell samples is a fundamental step. Quality preparation paves the way for a more successful sorting outcome. Initially, researchers must ensure they have a homogenous cell suspension. This requires meticulous handling to avoid damaging cells or altering their properties.
Key considerations include:
- Cell source: Choosing the right biological source is essential. Whether the cells are harvested from blood, tissue, or cultured lines, proper techniques must be applied for efficiency.
- Cell concentration: The concentration needs to be just right—too few cells may lead to poor yields, while too many can cause clumping and hinder the sorting process.
- Buffer solutions: Utilizing appropriate buffer systems is likewise critical. Buffers help maintain optimal pH and ion concentration, creating an environment conducive to cell viability.
Once these factors are set in place, it becomes easier to label cells for the subsequent sorting process.
Application of Magnetic Fields
The application of magnetic fields in MACS is where the actual ‘magic’ happens. Once the cells are properly labeled with magnetic beads, a magnetic field is introduced to the system, allowing for selective separation. This step is key to exploiting the inherent properties of the labeled target cells.
Considerations during this stage include:
- Field strength: The strength of the magnetic field impacts cell separation efficiency. Optimizing this can lead to thorough absorption of labeled cells to the magnet, making the process more effective.
- Magnet design: Different magnets can have varying designs which influence the movement and positioning of cells. Researchers may select specific magnet types, from simple hand-held devices to larger automated systems, depending on their requirements.
- Duration of exposure: The length of time cells are exposed to the magnetic field is also vital. Adequate contact time ensures complete attachment of magnetic beads to their targeted cells, thus yielding better isolation.
Through these tailored applications of magnetic fields, scientists can efficiently channel desired cell populations while leaving other types behind for further analysis.
Recovery of Target Cells
Following the application of magnetic fields, the recovery of target cells is the final step that pulls it all together. This is when the isolated cells are extracted from the mixture, marking an important transition from sorting to application.
Important factors in cell recovery include:
- Gentle elution techniques: Investment in gentle methods for eluting the target cells from the magnetic field is essential. Techniques should avoid stress to the cells, ensuring their viability post-recovery.
- Purity checks: It’s advisable to perform checks on the purity of the recovered cells. This can be done via flow cytometry or other suitable analytical methods to confirm the efficacy of the sorting process.
- Further processing: Depending on the next stages in research or clinical applications, further processing or analysis of the sorted cells may be warranted. Clearly laying out these next steps can streamline workflow and ensure effectiveness during subsequent experiments.
In summary, the operational methodologies of MACS dictate the quality and effectiveness of the sorting process. Understanding the critical aspects of sample preparation, magnetic field application, and cell recovery not only enhances the viability of the sorted cells but also maximizes the potential applications of MACS technology across various scientific fields.
Advantages of MACS Over Traditional Techniques
The realm of cellular biology has witnessed profound advancements thanks to various innovative techniques. Magnetic Activated Cell Sorting (MACS) is among the frontrunners, but why is it perceived as superior to traditional methods? To illustrate its value, consider four specific advantages: specificity in cell sorting, rapid processing times, and enhanced viability of sorted cells.
Specificity in Cell Sorting
When it comes to cell sorting, the need for precision cannot be overstated. MACS offers a remarkable edge here. Traditionally, methods such as flow cytometry rely heavily on fluorescent markers for identification, which can sometimes yield ambiguity due to overlapping signals.
With MACS, magnetic beads are coated with antibodies specific to target cell types. This means only the desired cells stick to the beads and can be isolated. As a result, the specificity in MACS ensures that contaminants are far less likely to compromise the outcome of experiments, especially vital in research settings involving distinct cell populations, like stem cells or immune cells.
Moreover, this precision reduces the time and resources spent on troubleshooting and optimizing workflows, thus reinforcing the argument for MACS being the go-to choice in modern laboratories.
Rapid Processing Times
Speed is often as critical as accuracy in research. Traditional purification methods can be an all-day affair, dragging on for hours or even days before researchers see any results. MACS changes that narrative by streamlining cell separation processes significantly.
The operational design of MACS allows researchers to complete sorting in a fraction of the time compared to conventional methods. Most MACS protocols can yield results in under an hour, making it easier to cycle through samples and increasing throughput dramatically.
Rapid processing not only enhances productivity; it can also be pivotal in time-sensitive experiments, such as those involving live-cell assays or detecting early cellular responses to treatments.
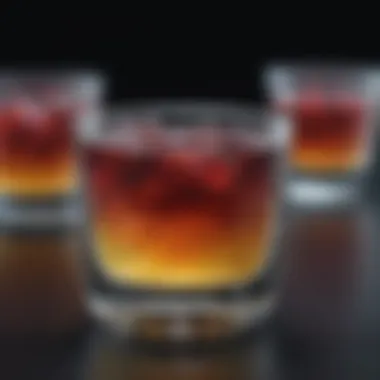
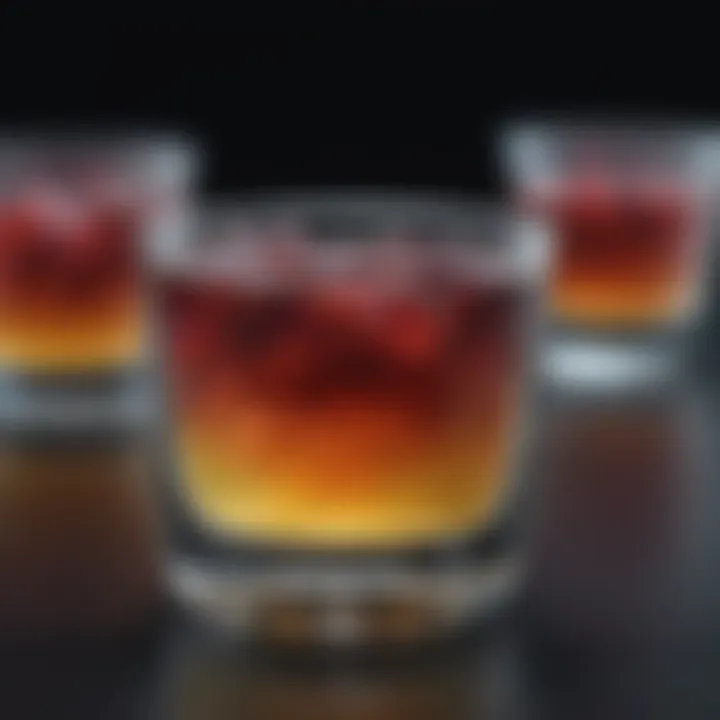
Enhanced Viability of Sorted Cells
One of the most notable benefits of MACS is how it preserves the integrity of sorted cells. Traditional techniques can often be much harsher, exposing cells to stress that can render them unviable for further research or clinical applications.
MACS, on the other hand, employs a gentle separation process. The magnetic fields used in MACS allow for a more non-invasive approach, meaning that target cells are less likely to sustain damage. This results in higher post-sorting viability rates, preserving not only cell function but also phenotype.
Higher viability translates into more reliable experimental results, particularly when dealing with sensitive cell types such as T-cells or hematopoietic stem cells, which are crucial for effective therapies and research alike.
Applications of MACS Across Disciplines
The versatility of Magnetic Activated Cell Sorting (MACS) technology is palpable across various fields. Understanding its applications underscores not only the technique's significance in biological research but also its transformative potential in clinical practices. As researchers endeavor to isolate specific cell types for study, MACS surfaces as an invaluable tool, simplifying processes and enhancing outcomes. Let's explore how MACS finds its footing in key areas such as immunology, oncology, stem cell research, and hematology.
In Immunology Research
In immunology, the precision offered by MACS is akin to having a finely tuned instrument. Researchers often require specific cell populations to investigate immune responses, disease mechanisms, and therapeutic interventions. By leveraging the ability of MACS to target particular cell markers, scientists can enrich populations of immune cells such as T cells or dendritic cells from heterogeneous samples. This enriched population is crucial for understanding intricate immune interactions.
The impact of MACS in immunological studies includes:
- Focused Therapeutic Development: By isolating specific immune cell types, researchers can develop targeted treatments that precisely modulate immune responses.
- Enhanced Study of Disease Models: The purification of immune cells aids in creating more accurate models of diseases like autoimmune disorders and transplant rejection.
- Identification of Novel Biomarkers: MACS facilitates the exploration of cell surfaces, leading to the identification of new markers that help in diagnosing various immune-related conditions.
In Cancer Therapy
Cancer therapy has witnessed groundbreaking advancements through the application of MACS. Here, the technology allows for refinement in patient treatment protocols by isolating cancer stem cells or specific tumor-infiltrating lymphocytes. This is particularly significant in developing personalized medicine approaches that tailor interventions to individual patient needs.
Considerations in cancer therapy using MACS include:
- Selective Targeting: The ability to harness specific cell types that are responsible for tumor growth or immune evasion enhances treatment efficiency.
- Research Applications: Studies can illuminate the cellular microenvironment of tumors, leading to better strategies for combating malignancies.
- Therapeutic Coatings: MACS can also be used to modify therapeutic agents, allowing for direct targeting and minimal systemic effects.
In Stem Cell Research
The fascination with stem cells stems from their unique capabilities in regenerative medicine. MACS provides an effective method for isolating and characterizing stem cell populations, a crucial step in both fundamental research and clinical applications. By enriching stem cell populations, researchers can gain insights into differentiation pathways and lineage specifications.
Key aspects include:
- Quality Control: Isolated stem cells can be rigorously tested for their pluripotency and differentiation capabilities, ensuring only high-quality cells are used for further research.
- Streamlined Clinical Applications: With the ability to obtain specific types of stem cells efficiently, MACS supports the advancement of therapies aimed at tissue regeneration and repair.
- Exploration of Stem Cell Biology: Researchers can better understand the signaling and environmental factors that govern stem cell behavior through enriched populations.
In Hematology Laboratories
In hematology, MACS plays an essential role in analyzing and sorting various blood cell types effectively. From isolating specific leukocyte populations for further study to aiding in blood transfusion practices, the applications are wide-ranging.
The advantages in hematology include:
- Accurate Diagnostic Tools: By sorting blood components with precision, MACS enhances the accuracy of diagnostics, particularly in understanding diseases such as leukemia.
- Research Enhancements: Exploring the functional capacities of specific blood cells, such as platelets or erythrocytes, becomes more achievable with MACS.
- Improved Transfusion Strategies: Tailoring blood components through selective sorting can lead to better patient outcomes in transfusions and related therapies.
"MACS not only simplifies but also revolutionizes how we approach cellular research and therapy. Its applications in diverse fields illustrate its significance in modern science."
The continuing integration of MACS into varying research avenues is a promise of more refined, effective strategies to confront biological challenges. As we look to the future, the breadth of its applicability shines brightly across disciplines, solidifying its standing as a cornerstone in cellular biology.
Challenges and Limitations of MACS Technology
Despite its many benefits, Magnetic Activated Cell Sorting (MACS) isn’t without its challenges. Understanding these limitations is crucial for researchers and clinicians who are considering adopting this technology. Identifying the hurdles which MACS encounters allows for better planning and implementation of this innovative cell sorting method. Here, we’ll dive into three significant challenges: cost implications, the potential for non-specific binding, and the technological advances needed for MACS to reach its full potential.
Cost Implications
The costs associated with implementing MACS can be a significant concern for many labs. While the technique can improve efficiency and cell viability, the initial investment is not trivial. The need for specialized equipment—such as magnetic separators and high-quality magnetic beads—adds up quickly. Additionally, the ongoing expenses like reagents and maintenance can pose a substantial financial burden, especially for smaller research facilities or start-ups.
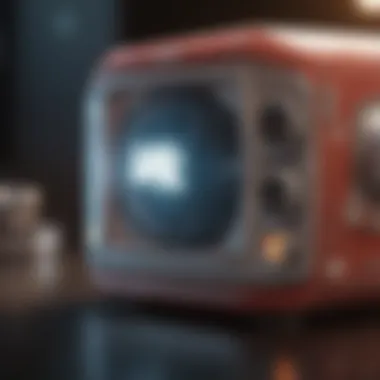
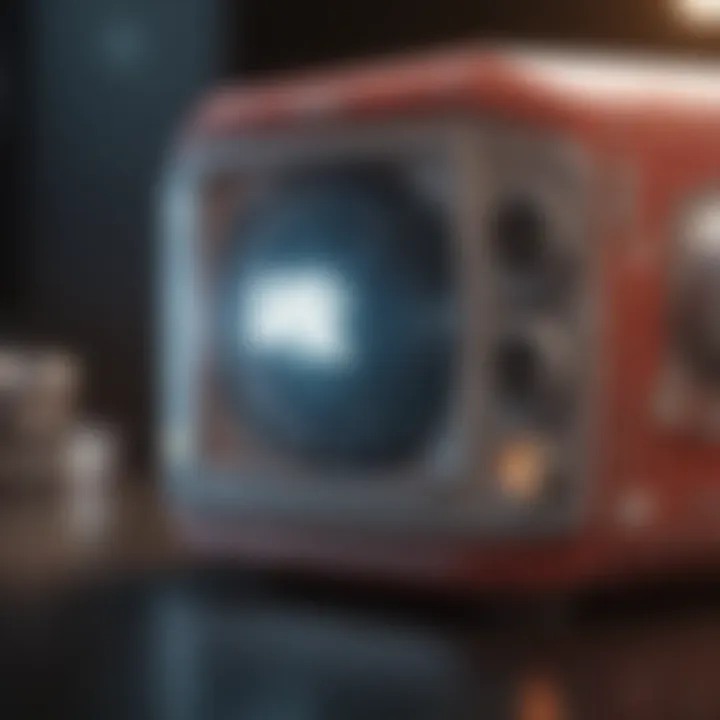
- Equipment costs: The upfront purchase price of MACS-compatible instruments and accessories can be prohibitive.
- Reagents: Magnetic beads that specifically target certain cell types are often priced at a premium, leading to increased costs per experiment.
- Training: Personnel may require training to ensure that they are familiar with MACS procedures, which could mean additional costs in both time and money.
It's evident that while MACS presents substantial advantages, financial resources can limit its accessibility and widespread adoption in various fields of research.
Potential for Non-Specific Binding
Another challenge that researchers face with MACS is the potential for non-specific binding of magnetic beads to unintended cell populations. This issue can lead to inaccurate cell separation and analysis, undermining the reliability of experimental results. Non-specific interactions can make it difficult to isolate pure populations, risking the integrity of downstream applications, such as gene expression analysis or therapeutic development.
- Complex mixes: When dealing with heterogeneous cell suspensions, distinguishing between desired and undesirable cell types can become convoluted.
- Optimization issues: The protocol optimization for each new cell type can be time-consuming and require multiple trials, adding complexity to the workflow.
- Impact on data quality: Inaccurate sorting can result in skewed data interpretation, which may lead to erroneous conclusions and hinder progress in research.
In battling this challenge, efforts must be made to refine labeling strategies and improve reagents that minimize non-specific binding and increase the fidelity of the sorting process.
Technological Advances Required
Despite the innovative nature of MACS, it’s clear that further technological advancements are necessary to maximize its efficiency and effectiveness in cell sorting applications. These improvements could greatly broaden MACS’s usability and address some of the limitations discussed.
- Miniaturization: The development of compact and portable devices could facilitate the use of MACS in fieldwork, making it more accessible in various settings, from clinics to rural areas.
- Enhanced detection methods: Improving the specificity of detection techniques—perhaps through the integration of advanced imaging methods—could greatly improve sorting accuracy.
- Real-time monitoring: Incorporating real-time feedback systems may help in monitoring cellular interactions during the sorting process, thereby adjusting parameters on-the-fly to enhance purity.
As the field continues to evolve, addressing these technological constraints presents an opportunity to further legitimize MACS as a cornerstone technology in cell biology research and clinical applications.
"While few techniques can claim to be without their pitfalls, understanding and addressing the challenges of MACS is key to unlocking its full potential."
Future Prospects of MACS in Research and Clinical Practice
The field of cell sorting and analysis is constantly evolving. Magnetic Activated Cell Sorting (MACS) stands out due to its ability to streamline the isolation of specific cell populations, paving the way for new research avenues and clinical applications. As we look ahead, the integration of MACS techniques into various research methodologies is not just beneficial but essential for advancing our understanding of cellular behaviors and interactions.
One of the most significant trends in the future of MACS is the integration with other cutting-edge technologies. This synergy can amplify the advantages that MACS already provides, allowing researchers to push the envelope further. For instance, the pairing of MACS with flow cytometry can yield higher resolution data on distinct cell markers and characteristics. This combined approach offers precision that might have escaped earlier methodologies. As labs adopt such integrative strategies, we could witness a surge in breakthroughs within disciplines such as immunology or regenerative medicine.
“Integrating MACS with other technologies will be a game-changer in both research and clinical settings, enhancing the accuracy and efficiency of cell isolation.”
Integrating MACS with Other Technologies
The push to integrate MACS with other techniques, particularly genomics and proteomics, represents a significant advancement in personalized medicine. By harnessing data from genetic analyses alongside cell profiles obtained via MACS, researchers can achieve insights that are tailored more explicitly to individual patients' medical profiles. This integration holds substantial promise for the development of targeted therapies and the customization of treatment regimens.
Moreover, the rise of artificial intelligence in biomedical research could result in more intricate data analytics, leading to improved interpretations of cell populations sorted via MACS. With AI algorithms capable of processing vast amounts of data rapidly, researchers could uncover patterns and insights that were previously hidden, thus accelerating discoveries in disease mechanisms and treatment effectivity.
Expanding Applications in Personalized Medicine
The need for personalized approaches in medicine couldn't be more pertinent today. MACS is uniquely positioned to contribute to this effort through its flexibility and efficiency in isolating various cell types relevant to patient-specific treatments. For example, the adoption of MACS in regenerative medicine can help in gathering a population of stem cells from a patient's tissue sample.
In hematology, applying MACS to sort specialized white blood cells might advance our approach to treatments for leukemias or lymphomas, enhancing both prognosis and patient outcomes. Furthermore, using MACS in combination with next-generation sequencing would allow us to map the genomic landscape for isolated cells, paving the way for personalized therapies that target specific genetic abnormalities in patients.
Epilogue
The conclusion of this article serves a pivotal role in synthesizing the nuanced discussions surrounding Magnetic Activated Cell Sorting (MACS). It encapsulates the essentials highlighted throughout— from the fundamental principles of MACS technology, its operational methodologies, to its expansive applications across various scientific arenas. These insights are not merely academic; they underscore MACS's significance in advancing modern biomedical research and clinical applications.
Key elements of this conclusion include:
- The transformative impact of MACS: This technique is fundamentally altering how researchers approach cell analysis, facilitating the precise separation of desired cell populations.
- Enhanced research capabilities: The application of MACS enhances scientists' ability to conduct in-depth analyses in fields like immunology and stem cell research, where specificity is crucial.
- The pathway ahead: While we discussed challenges and limitations, the conclusion emphasizes the promise MACS holds for integrating with emerging technologies, surpassing its current boundaries, and thereby enhancing personalized medicine.
"The role of MACS isn't just in cell sorting; it's in redefining what we can achieve with cellular research in the era of precision medicine."
This culmination reiterates the essence of MACS by showing its relevance to contemporary science and research. By synthesizing information from previous sections, the conclusion draws a comprehensive picture of how such a technique enriches our understanding, paving the way for future innovations.
Summary of Key Points
- Transformation of cellular biology: MACS offers an innovative way to isolate specific cell types, altering traditional methods.
- Operational advantages: The speed and viability of sorted cells significantly enhance research outcomes.
- Cross-disciplinary applications: Extending beyond immunology to fields like regenerative medicine, cancer therapy, and hematology.
- Addressing existing challenges: Awareness of cost and technological limitations reinforces the need for ongoing development and integration with other methodologies.
The Role of MACS in Contemporary Science
Magnetic Activated Cell Sorting plays a crucial role in the evolution of contemporary science. It not only highlights advances in cell analysis but also enriches our understanding of complex cellular interactions in health and disease. MACS provides a framework that supports innovations in personalized medicine, a field that requires precise cell targeting and manipulation based on individual patient's needs.
As researchers aim for more detailed insights into cellular behavior, the ability to sort cells with high specificity through MACS technology becomes not just beneficial but essential. This technology will likely drive forward the next generation of biomedical research and therapeutic strategies, pushing boundaries beyond what was once thought possible.