Electrical Channels: Exploring Biological and Technological Impact
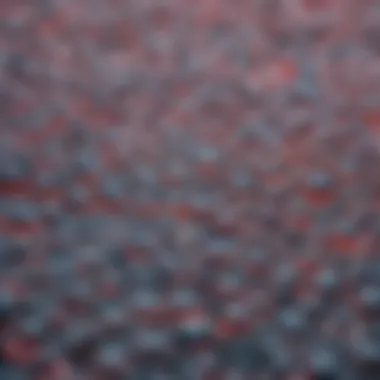
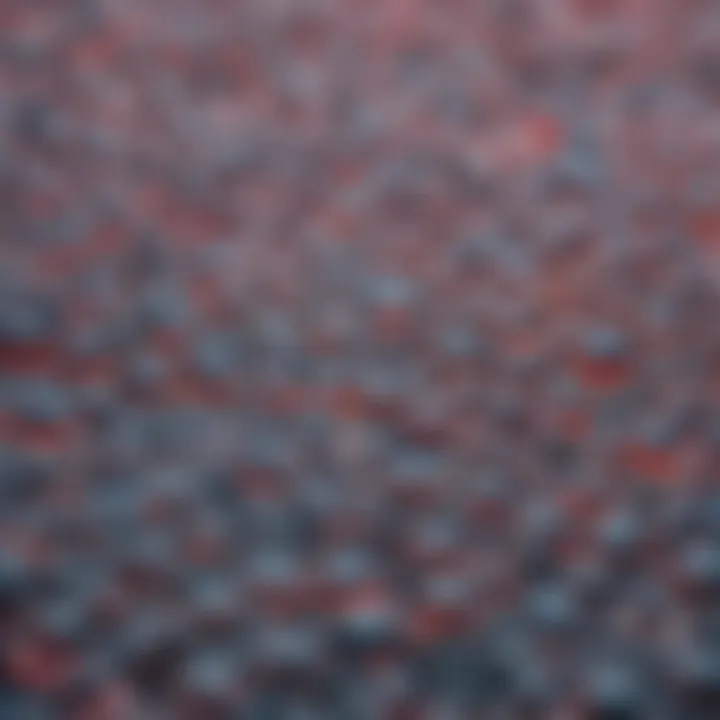
Article Overview
Electrical channels are more than just a subject of academic curiosity; they are central to both biological functions and modern technological innovations. To fully grasp their essence, the journey begins with an examination of their fundamentals, extending into diverse applications that can shape the future of both science and engineering. By exploring how electrical channels operate, how they differ across systems, and the burgeoning research in this field, we intend to provide a thorough understanding that appeals to scholars and practitioners alike.
Summary of Key Findings
- Electrical channels facilitate the movement of ions, playing a crucial role in cellular processes in biology, notably in neural activities.
- In the realm of technology, electrical channels are pivotal in devices like transistors and diodes, which are foundational components in circuit design.
- Recent advancements have integrated biotechnological innovations with nanotechnology, leading to more effective and efficient channel designs.
- Future developments hint at a symbiotic relationship between biological modeling and electrical engineering, paving the way for enhanced devices and therapeutic techniques.
Research Objectives
The primary goal of this article is to:
- Explore the different types and mechanisms of electrical channels across biological and technological realms.
- Analyze recent research trends that indicate the direction in which this field is heading.
- Discuss the practical implications of these findings in areas such as neuroscience and electrical engineering, looking to both current applications and future uses.
Key Results and Discussions
Main Findings
The exploration of electrical channels has unearthed several compelling insights:
- Types of Electrical Channels: There are various types of electrical channels, including voltage-gated, ligand-gated, and mechanically-gated channels, each serving distinct functions in biological systems.
- Technological Applications: In electronics, channels are categorized into analog and digital, crucial for the design of microchips and circuits. Their efficiency and design can dramatically influence device performance.
- Recent Advances: Innovative research, such as the development of smart materials and organic semiconductors, demonstrates how the duality of electrical channels can bridge biology and technology effectively.
Implications of Findings
Understanding electrical channels opens up avenues of research that can lead to significant advancements:
- In neuroscience, insights into ion channel behavior can bolster medical treatments for neurological disorders.
- For engineering fields, refining electrical channels contributes to more efficient power systems and faster, more reliable electronic devices.
- The interdisciplinary nature of electrical channels suggests a future where biology and technology converge, offering unprecedented opportunities for solving complex problems.
"The interplay between natural and artificial electrical channels has only just begun to reveal its full potential. Embracing this unity could lead us to groundbreaking developments in both health care and technological innovation."
Through this analysis, the goal is to not only illuminate the fundamental principles behind electrical channels but also to establish a clear connection to their practical applications, especially as we push the boundaries of innovation and understanding.
Foreword to Electrical Channels
Understanding electrical channels is like peeling back the layers of an onion; there’s always more beneath the surface. These channels play a pivotal role not just in our everyday gadgets but also in the very fabric of life itself. They act as conduits, allowing the flow of electrical signals that are essential for both communication in electronics and biological processes in organisms.
A profound grasp of electrical channels is invaluable for students, researchers, and professionals alike. It's not merely about comprehending electrical flow; it’s about recognizing their function in energy transfer, signal transmission, and the intricate dance of molecules in living systems. Imagine a world without these tiny yet powerful pathways. It would be a reality devoid of communication between neurons, halting our ability to think and react. In the tech realm, devices wouldn't function as they do without semiconductor elements and transistors, whose operations hinge on the properties dictated by electrical channels.
Definition and Basic Concepts
Electrical channels can be viewed as specialized pathways that enable the movement of electric charges. These pathways can be found in various forms — from biological ion channels in cell membranes to semiconductor materials used in computer chips. In biological terms, an ion channel is a protein structure that can open or close to control ion flow across membranes, impacting cellular activities. In electronics, a channel might refer to the conductive pathways used in semiconductor devices, affecting everything from signal processing to logic operations.
To break it down further, several key characteristics define these channels:
- Conductance: How easily electrical current can flow through the channel. Higher conductance means lower resistance.
- Selectivity: Some channels are picky about which ions or electrons can pass through, allowing for precise control in applications.
- Gating Mechanisms: Many channels open or close in response to specific stimuli, such as voltage changes or chemical signals, crucial in biological signaling.
Historical Context
The journey of electrical channels is deeply woven into the history of science and technology. In the late 19th century, discoveries in electrochemistry and the advent of the electron theory laid the groundwork for understanding how electrical flow works in both biological systems and man-made devices.
The initial insights into ion channels emerged with the introduction of the Nernst equation, which described ion concentrations and their impact on membrane potential. This was a game-changer for neurobiology, allowing scientists to explain how neurons transmit signals.
Fast forward to the 20th century, the field of electronics experienced an explosion of innovation. The invention of transistors revolutionized how electrical channels were designed and utilized in circuits, significantly enhancing computational power and efficiency.
In both domains, ongoing research continues to unlock mysteries and improve applications, ensuring that electrical channels remain at the core of scientific and technological advancement.
"Electrical channels are not merely conduits for energy; they are gateways that unlock the secrets of life and technology."
Types of Electrical Channels
Electrical channels play a crucial role in both biological systems and technological applications, offering a variety of functionalities essential for different processes. Understanding the types of electrical channels helps us appreciate their unique mechanisms and how they contribute to their respective fields. Each category, whether it be ion channels or semiconductor channels, carries significant implications for health, innovation, and research.
Ion Channels in Biological Systems
Ion channels are integral membrane proteins that mediate the flow of ions across cell membranes. They are vital for processes such as nerve impulse transmission, muscle contraction, and cellular signaling. Let's take a closer look at the different types of ion channels and their importance.
Voltage-Gated Ion Channels
Voltage-gated ion channels are a particular type that opens or closes in response to changes in membrane potential. This characteristic is vital for generating action potentials in neurons. The key trait of voltage-gated channels is their response to the electrical charge across the membrane, making them critical for rapid signaling.
The unique feature here is the presence of voltage sensors within the channel structure. This allows them to respond swiftly, facilitating quick communication in the nervous system and muscle tissues. However, not all voltage-gated channels operate perfectly in every situation. For instance, certain diseases, like epilepsy, can arise when these channels malfunction, underscoring their complexity and importance in maintaining cell function.
Ligand-Gated Ion Channels
Ligand-gated ion channels differ from their voltage-gated counterparts by requiring specific molecules—called ligands—to bind to them before they can open. This property helps in mediating neurotransmitter actions at synapses. A key characteristic of ligand-gated channels is their specificity to certain ligands, making them fundamental in neuronal communication.
A unique feature of these channels is that their activation often leads to significant downstream effects, including muscle contraction and hormone release. However, this specificity can also be a double-edged sword. If the ligand is present in excess, it can lead to hyperactivity or other imbalances, which can have detrimental effects on overall health.
Mechanosensitive Ion Channels
Mechanosensitive ion channels are fascinating in that they open in response to mechanical deformation of the cell membrane. This characteristic provides a bridge between physical stimuli and cellular responses. A notable characteristic of these channels is their ability to convert mechanical signals—like pressure or stretch—into electrical signals.
Their unique feature is their involvement in essential physiological processes such as touch sensation and the regulation of blood pressure. However, this responsiveness can sometimes lead to issues. For example, when mechanically-induced stress becomes too much, it can result in cellular damage, highlighting the need for precise balance in this mechanism.
Semiconductor Channels
Semiconductor channels are pivotal in electronic devices, where they enable repeated cycles of conduction and insulation. Understanding these channels helps in exploring various applications in modern technology, such as computers, smartphones, and renewable energy systems. Let’s examine the primary types found within this category.
Field-Effect Transistors
Field-effect transistors (FETs) are essential in controlling electrical signals. Their ability to modulate current flow through an electric field makes them advantageous for amplifying signals. A key characteristic of FETs is their high input impedance, which helps in minimizing power loss.
The uniqueness of these devices lies in their efficiency and versatility—FETs are widely used in everything from signal processing to microprocessor applications. However, they can be sensitive to factors like temperature variations, which can affect their performance.
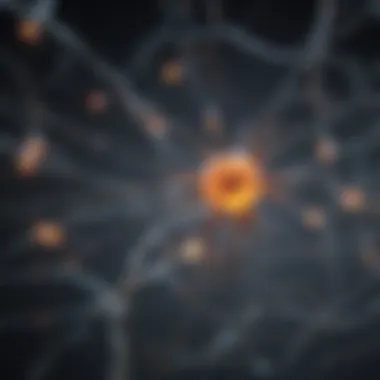
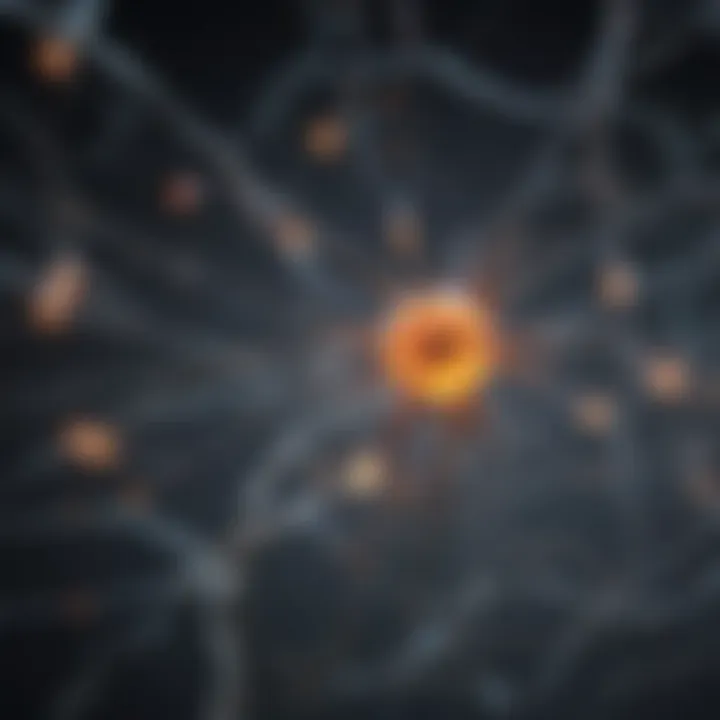
Junction Transistors
Junction-transistors work by utilizing semiconductor junctions to control current. They are notable for their ability to amplify signals, making them fundamental in developing various electronic applications. Their key characteristic is the junction formed between different types of semiconductors, typically p-type and n-type.
Their unique feature is their robustness, allowing for reliable performance across various conditions. However, this is not without downsides; poor design or quality can lead to thermal runaway, resulting in device failure.
Quantum Dots
Quantum dots are semiconductor particles that are small enough to exhibit quantum mechanical properties. This unique aspect enables them to be utilized in advanced technologies, particularly in developing high-resolution displays and photovoltaic cells. A notable characteristic of quantum dots is their size-tunable light emission, which allows for versatile applications in electronics and photography.
While these benefits are significant, there are challenges as well. The synthesis of quantum dots can be expensive and complicated, limiting their widespread application.
Mechanisms of Functionality
Understanding the mechanisms of functionality of electrical channels is a pivotal aspect of this exploration. It is within this domain that the intricate workings of electrical channels illuminate the paths of both life and technology. Here, we delve into electrical properties and channel gating mechanisms, which are foundational to how these channels operate in varying conditions.
Electrical Properties
Electrical properties such as conductance, resistance, and capacitance play vital roles in the functioning of electrical channels, affecting everything from cellular signaling to the performance of electronic devices.
Conductance
Conductance is fundamentally about how well a channel allows the flow of electric current. It is a key characteristic that defines a channel's efficiency in conducting electricity. A higher conductance means less resistance to current flow, making it an indispensable property for both biological and electronic systems.
In the frame of biological systems, high conductance in ion channels supports rapid signaling in neurons. This allows for faster communication across synapses, essential for reflexes and sensory processing. Conversely, in semiconductor devices, such as those found in smartphones, effective conductance facilitates quick data processing and transmission.
However, there are disadvantages; too much conductance can lead to leakage currents, which may introduce inefficiencies in the system.
Resistance
Resistance, on the other hand, refers to the opposition to current flow within a channel. This property is crucial as it allows for current regulation. For example, in nerve cells, the resistance of certain ion channels ensures that action potentials can be precisely controlled, contributing to the overall signal stability in neuronal communication.
In terms of electronics, appropriate resistance levels help in managing power consumption. While resistance is necessary for preventing external interference in circuits, too much resistance can slow down signals, presenting a challenge for high-speed applications.
Capacitance
Capacitance measures how much electric charge a channel can store. This property is especially important in the context of biological membranes, where capacitance affects signal propagation. In technology, capacitance is a defining characteristic of components like capacitors used in filtering applications.
A unique feature of capacitance is its role in stabilizing voltage levels. It buffers sudden changes in voltage, ensuring a smoother operation for both living cells and electronic circuits. However, high capacitance can lead to slower response times, which may limit its applications in high-speed environments.
Channel Gating Mechanisms
Channel gating mechanisms determine how and when channels open or close, controlling ion flow and electrical signals. These mechanisms include responses to chemical triggers, physical changes, and biochemical signals, each playing a crucial role in both biological and technological contexts.
Chemical Triggers
Chemical triggers, like neurotransmitters, bind to specific sites on ion channels, inducing their opening. This rapid response ensures that signals can be transmitted across neurons almost instantaneously. Chemical gating is a predominant mechanism in synaptic transmissions.
On the tech side, similar principles apply in various devices where components respond to specific environmental changes, creating a reaction to stimuli. However, reliance on chemical triggers can lead to delays due to reaction times resulting from biochemical interactions.
Physical Changes
Physical changes refer to alterations in channel structure that can open or close gates. For instance, mechanical stretching can activate stretch-sensitive ion channels in cells, a critical process in sensing touch or pain. This physical gating is essential for the bodily response to the surrounding environment.
In technology, physical mechanisms are explored in various devices, enabling adaptability to varying conditions. However, countless external variables can affect this physical mechanism, leading to instability or failure in operational settings.
Biochemical Signals
Biochemical signals represent another layer of complexity in channel gating. They can determine channel behavior based on cellular metabolic states, providing an additional control layer in biological systems. For example, in muscle cells, energy availability can influence the opening of ion channels, impacting muscle contraction.
In electronics, understanding how signals influence component behavior can yield designs that are more efficient and responsive. Nonetheless, this also leads to complexities in designing circuits where such dynamic responses are required.
In summary, the mechanisms of functionality of electrical channels are interwoven through various aspects that define their role across multiple fields. Understanding conductance, resistance, capacitance, and the gating mechanisms provides a richer comprehension of both biological processes and technological innovations.
The Role of Electrical Channels in Neuroscience
Electrical channels are fundamental players in the complex network of the nervous system. These structures not only facilitate communication between neurons but also influence a plethora of physiological functions across the body. Understanding the role of electrical channels in neuroscience is crucial for grasping how signals are transmitted, processed, and ultimately transformed into actions or responses.
One key aspect of electrical channels is their involvement in action potentials, the rapid rise and fall of membrane potential that constitutes a signal within nerve cells. This process begins when a neuron receives input from other neurons or sensory receptors. Upon reaching a certain threshold, voltage-gated sodium channels open, causing a sudden influx of sodium ions. This event propels the action potential down the axon and ultimately leads to communication with other neurons, muscles, or glands. The efficiency and speed of this signaling depend heavily on the proper functioning of these channels, making them vital for rapid responses, as seen during reflex actions.
Moreover, electrical channels govern signal transmission quality. They can modulate the frequency and timing of impulses sent through synapses, dictating how information is relayed and interpreted by the nervous system. A variation in channel activity can lead to differences in how stimuli are perceived—for instance, subtle changes can translate to varying sensations of pain or touch. Thus, the precise regulation of these electrical pathways is essential to maintaining homeostasis and cognitive functions.
Action Potentials and Signal Transmission
In neuroscience, action potentials serve as the primary means of information transfer. When discussing them, we enter the realm of excitable cells, primarily neurons and muscle cells, which can generate these electrical signals. The sequence of changes in membrane permeability is dictated by the opening and closing of ion channels. This sequential opening is typically characterized by phases:
- Resting Phase: The neuron rests, and sodium channels are closed, maintaining a negative internal environment.
- Depolarization: Initiated by stimuli, sodium channels open, causing an influx of sodium ions, leading to a positive charge inside the cell.
- Repolarization: After reaching peak depolarization, sodium channels close, and potassium channels open, allowing potassium to flow out and restore the negativity.
- Hyperpolarization: The membrane potential dips below resting level before stabilizing, clearing the way for the next action potential.
This finely-tuned process allows for the quick transmission of signals over long distances and helps coordinate complex functions like movement, thought, and reflexes. Furthermore, myelin sheath—a fatty substance wrapping around axons—enhances signal transmission by insulating the axon, enabling ions to propagate signals more efficiently through saltatory conduction.
Neurological Disorders Related to Ion Channels
Dysfunction in electrical channels can lead to significant health implications and is often at the core of various neurological disorders. These disorders, broadly termed channelopathies, arise from genetic variations, environmental factors, or even trauma that alter the way ions flow through these channels.
Some notable examples include:
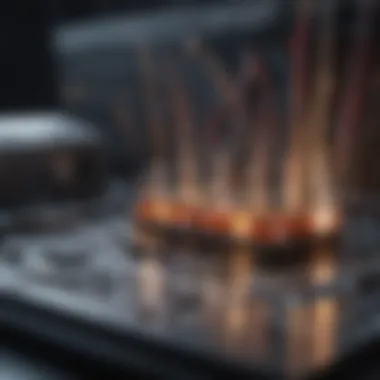
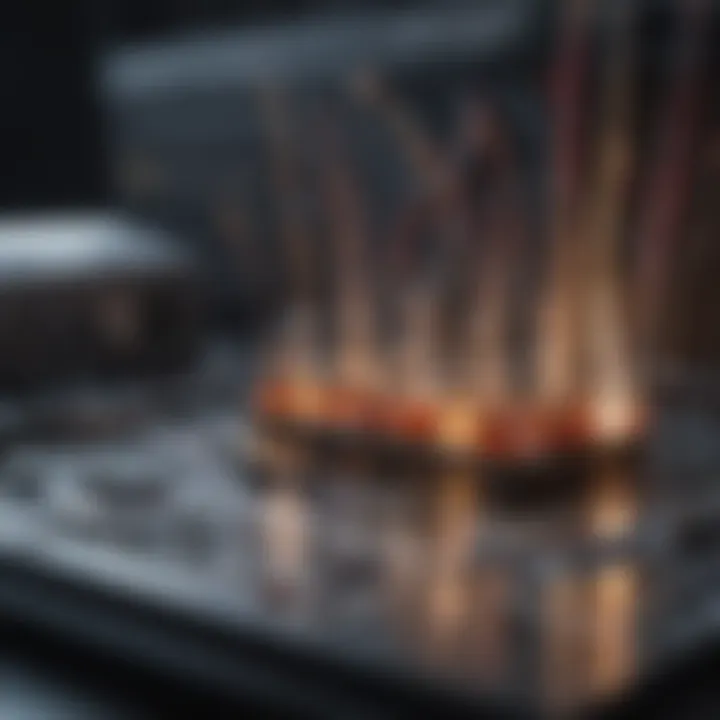
- Epilepsy: Characterized by seizures, certain types of epilepsy arise from mutations in sodium channels, leading to abnormal electrical activity.
- Migraines: Changes in ion channel activity can trigger prolonged headaches; calcitonin gene-related peptide (CGRP) has been implicated in this process.
- Cystic Fibrosis: This is a classic example affecting the CFTR ion channel, leading to severe respiratory issues due to a buildup of thick mucus.
Moreover, research continues to uncover the intricate relationship between electrical channels and other conditions, such as cardiac arrhythmias, where disruptions in ion channel function can affect heart rhythm. The ongoing study into these disorders underpins the increasing recognition of the critical role that electrical channels play in health and disease, propelling scientific interest in possible therapies and treatments that target these pathways.
The importance of electrical channels in neuroscience cannot be overstated; they are the linchpins in both normal and abnormal neuronal communication.
In summary, electrical channels serve as vital conduits in the delicate balance of neurosystem functionality. Their roles extend beyond mere conduits of information, influencing overall health and neurological integrity. Understanding these channels is essential for educators, students, researchers, and professionals aiming to innovate in fields related to neuroscience.
Technological Applications
In the realm of both biology and engineering, electrical channels have carved out a niche that is indispensable. They act as gateways, facilitating various processes that range from cellular communication to signal transmission in electronic devices. Understanding these applications broadens the horizon of innovation, shaping both present and future technologies. The versatility of electrical channels means insights gained can drive advancements in fields such as nanotechnology, biomedicine, and circuit design, highlighting the necessity of integrating this knowledge into contemporary practices.
Use in Electronic Devices
Transistors in Circuit Design
Transistors are the backbone of modern electronic design. These devices manage the flow of current and are integral in amplifying and switching signals. Their primary feature—ability to act as an on/off switch—makes them highly effective in circuits, which rely on binary states for function.
Transistors offer a key advantage: miniaturization. As circuits grow smaller, so too do the transistors. This has led to the development of compact devices that dominate today’s tech scene, from smartphones to embedded systems in automobiles. However, this diminutive aspect brings forth challenges, such as heat dissipation and material limitations. It's crucial to maintain a delicate balance; go too small, and reliability may be compromised.
Role in Integrated Circuits
Integrated circuits (ICs) have revolutionized technology by packing thousands of transistors into a single chip. This has simplified wiring and revolutionized manufacturing processes. The most significant characteristic of ICs is their ability to achieve high performance while consuming minimal power, which is essential in portable electronics.
One unique feature of ICs is their capability to integrate various functions—like computing, switching, and signal processing—onto a single chip. This not only saves space but also enhances performance. However, as with transistors, there are downsides. Manufacturing complexity increases, which can drive costs, and any defect during production can render an entire chip useless.
Comparative Analysis with Other Components
When evaluating electrical channels, it's essential to consider other electronic components like diodes and resistors. Transistors excel in switching and amplification, while diodes serve more as one-way gates for current flow. Resistors limit current, thus controlling voltage.
One key point in this comparative aspect is the functionality. Transistors process analog signals, while diodes are geared toward rectification. Recognizing these distinctions allows engineers to select the appropriate components for specific applications. However, the use of different components together might introduce a level of complexity that could overcomplicate circuit design.
Advancements in Research and Development
Nanoscale Electrical Channels
Emerging research around nanoscale electrical channels has opened doors into new technologies. These miniaturized channels can manipulate electrons at levels previously thought impossible, leading to faster data processing and reduced energy requirements. The defining trait of nanoscale channels is their size; they operate on a scale much finer than traditional electronic components, leading to significant changes in performance metrics.
The primary challenge with nanoscale channels lies in manufacturing consistency. As sizes decrease, the tolerances tighten, which could lead to instability or variations in performance. Yet, successfully navigating this can lead to revolutionary changes in how we think about data processing and electronic design.
Biocompatible Channels in Medicine
In the medical field, biocompatible electrical channels are making waves. These channels can interface safely with biological systems without causing harm or rejection, making them invaluable for neuromodulation or drug delivery systems. The standout characteristic of biocompatibility is that they can function without disrupting the body’s natural processes.
One of the prominent features is their ability to promote cellular interaction, enhancing therapies aimed at nerve damage or chronic conditions. Still, these channels can face limitations in terms of durability and long-term reliability within a living organism, requiring ongoing research to improve their lifespan and performance.
Future Prospects in Technology
Looking ahead, the future of electrical channels appears bright. With continuous advancements, we anticipate leaps in efficiency and functionality, particularly in how they are integrated into everyday devices. A significant characteristic of this future is the potential for smart technologies that could allow devices to self-regulate based on user interaction and environmental stimuli.
However, with each innovation comes the concern over ethical implications and sustainability. As we push boundaries, ensuring responsible development and application is essential for addressing public concerns. The convergence of electrical channels with artificial intelligence and machine learning could reshape industries from healthcare to home automation, elevating the role of electrical channels beyond mere components to essential elements of modern life.
"In the ever-evolving landscape of technology, understanding electrical channels opens the door to innovative solutions and future breakthroughs."
Impact on Health and Medicine
The influence of electrical channels on health and medicine is profound and multifaceted. These channels do not merely exist as theoretical constructs; they serve critical functions in both biological systems and therapeutic interventions. Ion channels regulate various physiological processes, while their malfunction can lead to numerous health conditions. Understanding these dynamics provides a bridge between molecular biology and clinical applications.
In this section, we focus on two primary aspects:
- Medical Applications of Ion Channels: Recognizing how these channels are utilized in current medical practices could illuminate their significance.
- Channelopathies: Genetic Disorders: These disorders underscore the repercussions of electrical channel dysfunction within the human body. By exploring these topics, the role of electrical channels in health becomes clearer, revealing both the potential for innovative treatments and the challenges posed by genetic conditions.
Medical Applications of Ion Channels
Ion channels are not just cellular gatekeepers but also therapeutic targets. Their roles span across various branches of medicine, particularly in cardiology and neurology. For instance, anti-arrhythmic drugs often target voltage-gated sodium and calcium ion channels to correct aberrant electrical activity in the heart. This highlights their essential functions in maintaining regular heart rhythm and, by extension, overall cardiovascular health.
Likewise, in neuroscience, ion channels such as voltage-gated potassium channels are vital for repolarizing neurons after action potentials. Medications that modulate these channels can help treat epilepsy and other neurological disorders. A notable example is the use of lamotrigine, which acts on sodium channels, stabilizing neuronal membranes and preventing excessive electrical activity that leads to seizures.
Here are a few significant aspects of medical applications of ion channels:
- Drug Development: Targeted therapies are being developed to exploit ion channel functionalities, leading to more effective treatments with fewer side effects.
- Diagnostic Tools: Ion channel behavior can indicate certain disease states, offering diagnostic pathways through electrophysiological measurements.
- Personalized Medicine: Advances in genetic knowledge allow for tailoring treatments based on individual channelopathy profiles, enhancing treatment efficacy.
Channelopathies: Genetic Disorders
Channelopathies represent a spectrum of genetic disorders that arise from dysfunctional ion channels. The ramifications of these disorders can be severe, affecting cardiac rhythms, muscle function, and neuronal signaling. A well-known example is Long QT syndrome, which involves mutations in cardiac ion channels, leading to life-threatening arrhythmias.
Importantly, channelopathies can be challenging to diagnose and manage, as they exhibit a wide range of symptoms that may overlap with other conditions. Typically, they require a multidisciplinary approach for effective management. Here are some notable points concerning channelopathies:
- Genetic Testing: Identification of the specific mutations allows for better risk assessment and family screening.
- Symptom Management: Treatments may include lifestyle changes, beta-blockers, or even implantable devices in severe cases.
- Clinical Research: Continuous studies aim to understand the mechanistic pathways that underlie different channelopathies, contributing to the development of targeted therapies.
Understanding the intricacies of electrical channels within the context of health and medicine provides invaluable insights into both the mechanisms of disease and potential therapeutic strategies.
Through these explorations, it is clear that electrical channels are not isolated entities; they play critical roles in the physiological and pathological landscapes of the human body. This connectivity enriches our understanding of health and opens doors to innovative medical interventions.
Sustainability and Environmental Considerations
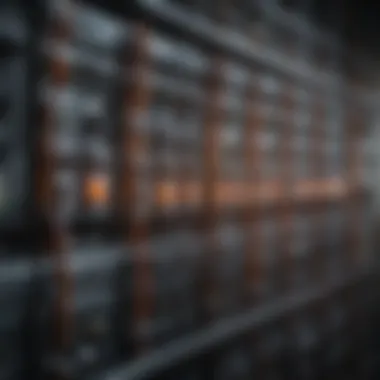
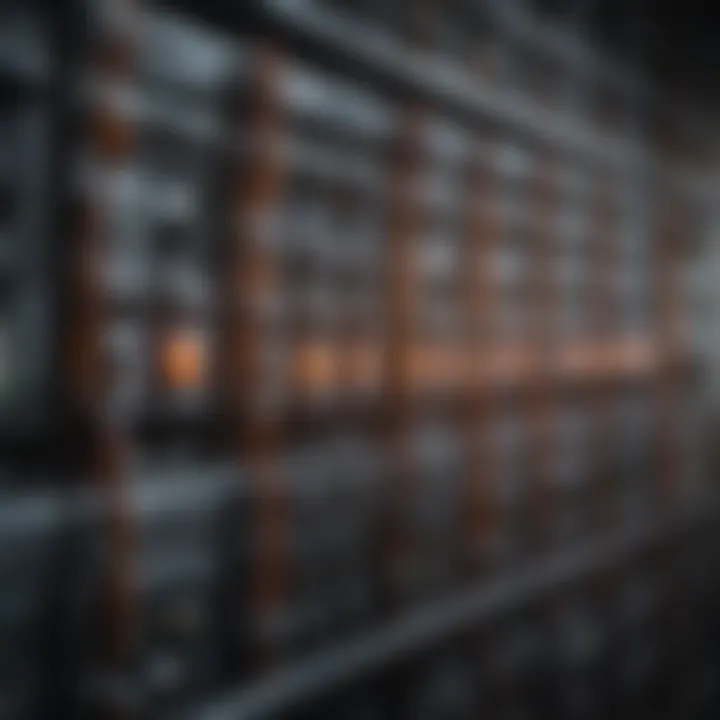
The discussion on sustainability and environmental considerations has become increasingly pertinent in contemporary discourse surrounding electrical channels. As technology evolves, it becomes paramount to evaluate how these electrical systems impact our planet and the responsibility of researchers and industry leaders in mitigating adverse effects. By addressing sustainability, we aim not only to improve our current practices but also to pave the way for future innovations that respect ecological balance and resource efficiency.
Eco-Friendly Alternatives in Electrical Channel Design
In pursuit of sustainable solutions, designers and engineers have been innovating eco-friendly alternatives to traditional electrical channels. Materials with lower environmental impact, such as bioplastics or recycled metals, are receiving attention as viable substitutes. Such choices not only reduce carbon footprints but also promote a circular economy where materials are reused and repurposed.
Examples include:
- Conductive Polymers: Utilizing these materials can lead to lighter and more flexible electrical components, reducing the energy needed for production.
- Green Construction Methods: Using less energy-intensive methods in fabrication can significantly lessen impact during the manufacturing phase.
- Integration of Renewable Energy: Designing channels that effectively integrate renewable energy sources can reduce reliance on fossil fuels, which is crucial for sustainable electronic platforms.
This shift towards greener alternatives does not simply benefit the environment; it also opens new avenues for performance enhancement and innovation. Companies adopting these practices often find themselves ahead of regulations and resonate well with conscientious consumers who are increasingly market-savvy about the ecological footprints of the products they consume.
Impact of Electronics on the Environment
The proliferation of electronics has undeniably contributed to several ecological issues. E-waste is one of the most pressing concerns; as devices become obsolete, they often end up in landfills, leaking harmful substances into soil and waterways. Furthermore, the extraction of raw materials needed for electronics often leads to habitat destruction and biodiversity loss.
The cycle of electronic production also demands a significant amount of energy, exacerbating the global energy crisis. It is essential to understand not just how these products are made but also how they affect the environment throughout their lifecycle.
Key factors to consider include:
- Resource Depletion: Mining metals and minerals can exhaust local resources, weakening ecosystems.
- Energy Usage: High energy demand during production contributes to greenhouse gas emissions.
- Toxic Waste: Improper disposal of electrical components can create hazardous waste that is detrimental to human health and the environment.
"We must consider the entire lifecycle of electrical channels. Sustainability transcends mere compliance; it demands innovation and conscientious design."
Ethical Considerations
Understanding the ethical implications surrounding electrical channels is of paramount importance, particularly as this field evolves and intersects with health, science, and technology. With multiple disciplines at play—ranging from neuroscience to biotechnology—it raises a host of questions about moral responsibilities and the potential consequences of research and development. These considerations lead to a greater awareness of how advancements can benefit society while minimizing harm to both individuals and the environment.
In the life sciences, ethical considerations touch on the appropriate use of ion channels in research and therapeutic interventions. Researchers must grapple with dilemmas about consent, data integrity, and the potential for misuse of technology. Ensuring that medical applications of ion channels—such as those used in pharmacology or genetics—are pursued with a clear focus on patient welfare is essential. This aligns with the ethical principle of beneficence, which emphasizes doing good while also avoiding harm.
A deeper dialogue regarding these challenges can inform the protocols in place for ongoing studies and practical applications. Such scrutiny encourages transparency and accountability, which ultimately contribute to the trust that underpins research in this area.
Ethics in Medical Research Related to Ion Channels
The interplay between medical research and ion channel studies brings forth a spectrum of ethical issues that cannot be ignored. The use of ion channels as therapeutic targets addresses numerous illnesses, ranging from cardiac arrhythmias to neurological disorders. In this regard, the quest for knowledge must be carefully monitored to ensure that it does not outpace ethical standards—particularly with respect to human trials.
When investigating potential treatments that manipulate ion channels, researchers must ensure that:
- Informed consent is obtained from participants, allowing them to make well-informed choices about their involvement.
- The risks of new therapies are thoroughly assessed and communicated effectively to participants, avoiding any surprises in the event of adverse effects.
- There is potential equity in the access to new therapies developed through research, which can prevent disparities in healthcare based on socioeconomic status.
Balancing the thirst for knowledge with ethical integrity is crucial. With ion channel research advancing rapidly, maintaining the highest ethical standards helps ensure that scientific progress aligns with public interest—serving all rather than just a select few.
Debates Surrounding Technology Use
As technology develops at breakneck speed, its implications on ethical grounds are a frequent topic of debate. When discussing electrical channels, particularly within semi-conductors and biocompatible materials, questions arise about how far society is willing to go in pursuit of innovation.
Issues under scrutiny include:
- Privacy: With increased data collected from medical devices that utilize ion channels, concerns regarding patient data privacy grow. These dimensions of ethics highlight the necessity for robust data protection regulations.
- Accessibility: There are fears that advanced technologies may create a divide, wherein only affluent demographics benefit from groundbreaking treatments. Equity in access is an essential conversation in ensuring that advancements reach all demographics.
- Accountability for Misuse: As with any powerful tool, the risk of misuse increases. Establishing guidelines for the ethical use of technology in both clinical and research settings is critical to mitigate potential harm.
"With great power comes great responsibility." This adage rings particularly true in the realm of medical research and technology. Emphasizing ethical considerations not only secures the integrity of scientific pursuits but also fosters a respectful dialogue around the implications of electrical channels in society.
In summary, it would be folly to ignore the ethical considerations surrounding electrical channels. Engaging these discussions not only guides responsible innovation but also ensures that advancements serve the greater good.
Future of Electrical Channels
The horizon looks promising for electrical channels, as they are on the brink of significant transformations that intertwine both biological insights and technological advancements. This exploration of the future doesn’t just reside in the realms of theory; it’s founded on tangible benefits and considerations that will shape industries and healthcare. The ability to understand how electrical channels function and how their manipulation can yield beneficial outcomes for society cannot be overstated. A few of the pivotal elements in this landscape involve emerging technologies, interdisciplinary applications, and the health implications of breakthroughs.
Emerging Technologies
In the rapidly evolving technological landscape, electrical channels are at the forefront of several emerging technologies. Here are a few key examples that encapsulate this growth:
- Nanochannel Systems: These tiny conduits can be used for drug delivery and biosensing. By controlling the flow of ions and molecules at a nano scale, researchers envisage a future where medical treatments become highly localized and efficient, minimizing adverse effects.
- Graphene-based Transistors: Thanks to their exceptional conductivity, graphene transistors have the potential to replace traditional silicon-based devices. This shift might lead to more powerful and faster electronic components, marking a substantial leap in processing capabilities.
- Synthetic Biology Applications: As scientists delve deeper into genetic engineering, there’s an increasing expectation for synthetic ion channels to emerge, which could be tailored for specific biological tasks. This breakthrough could lead to specialized cells that respond more effectively to environmental stimuli.
Moreover, the impact of such technologies will extend beyond electronics and into medical fields. For instance, innovations in electrical channel applications could facilitate real-time monitoring of health conditions using implanted devices.
Predictions for Advancements
The path forward for electrical channels is painted with predictions that could redefine various fields, especially neuroscience and electronics. Several anticipated advancements are noteworthy:
- Integration of Quantum Computing: The prospect of integrating quantum bits (qubits) within electrical channels is a hotbed for research. It is forecasted that advancements in quantum technology will necessitate new types of channels, leading to ultra-fast computations and storage solutions, vastly outpacing current capabilities.
- Smart Materials: The emergence of materials that can change their properties in response to stimuli—temperature, electric fields, etc.—will likely revolutionize the way electrical channels are designed. These materials can lead to breakthroughs in flexible electronics and wearable technology.
- Personalized Medicine: The trend toward personalized medical treatments will accompany advancements in our understanding of electrical channels. This will include tailored ion channel inhibitors for specific genetic conditions, leading to highly individualized therapeutic strategies.
- Enhanced Medical Imaging: Innovations in imaging technology could be tied closely to advancements in electrical channels, facilitating more detailed and accurate scans, allowing for early disease detection.
In summary, the future of electrical channels holds tremendous promise, with challenges and opportunities that invite deeper investigation. As researchers forge ahead in this domain, an amalgamation of biological understanding and cutting-edge technology is bound to uplift our scientific endeavors in ways we cannot yet fully comprehend.
"Innovation in electrical channels today will set the foundation for the breakthroughs of tomorrow."
Navigating these advancements will require a comprehensive understanding of the underlying principles governing electrical channels, further evidence of their pivotal role across various disciplines.
Finale
The exploration of electrical channels serves as a vital touchstone in our understanding of both biological processes and technological advancements. At its core, this article illustrates that these channels are not merely conduits for electricity; they embody a complex interplay of biological feedback loops and engineering innovations that drive many crucial functions in living organisms and electronic devices alike.
In recapping the critical themes discussed, it becomes clear that:
- Electrical Channels in Biology: We observed how ion channels facilitate rapid communication in nerve cells, enabling the firing of action potentials essential for bodily functions. They too play a significant role in the onset of various neurological disorders, underscoring their importance not only in health but also in potential clinical interventions.
- Technological Applications: The discussion highlighted how channels function in semiconductors, leading to advancements in electronic devices, and this realm won't stop innovating as research pushes boundaries in miniaturization and performance.
- Ethical and Environmental Considerations: We touched on the fine line between innovation and sustainability, balancing technological progress with ecological responsibility. Electrical channel design must consider not only efficiency but also its impacts on the environment.
Benefits of Understanding Electrical Channels: Grasping the mechanics of these channels provides insights that can translate into better clinical practices, enhanced electronic designs, and a spotlight on ethical considerations that govern research and application.
“Our grasp of electrical channels could one day revolutionize both medicine and technology, shaping a future where the line between the biological and technological may blur.”
In essence, this conclusion draws attention to the necessity of integrating knowledge from various disciplines to forge a path forward, where our enlightened understanding of electrical channels may inspire innovations that redefine what we deem possible.