In-Depth Insights on Calcium Imaging Analysis
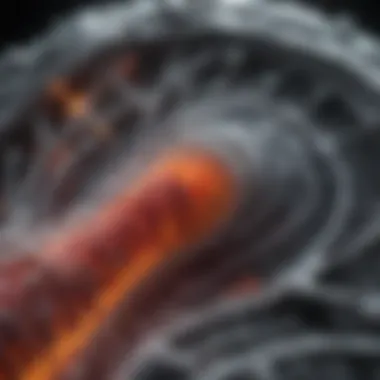
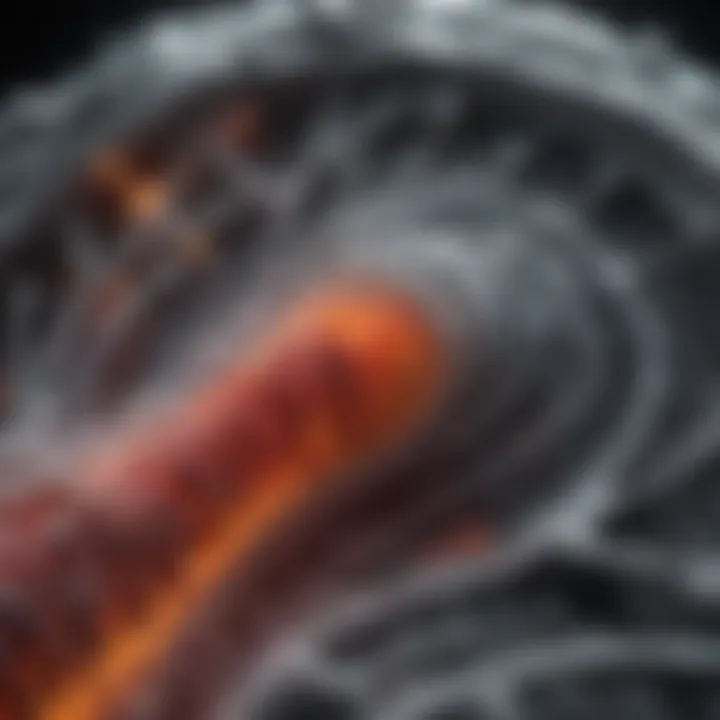
Intro
Calcium imaging analysis serves as a crucial tool in various scientific fields, particularly in neuroscience and cell biology. Through this technique, researchers can visualize and quantify calcium ions in living cells, facilitating a deeper understanding of cellular activities and interactions. In recent years, advancements in imaging technology have enhanced the capabilities of this method, making it more accessible and effective.
The complexity of analyzing calcium signals can, however, pose challenges. These include variations in signal intensity, noise, and data processing requirements. Therefore, a comprehensive exploration of this technique is essential for those involved in cutting-edge research. Understanding these elements is vital not only for researchers but also for students and educators who aim to grasp the fundamental concepts underlying calcium imaging analysis.
In the sections below, we will delve into the article overview, highlighting key findings and research objectives related to this important topic.
Prolusion to Calcium Imaging
Calcium imaging is a critical technique in the study of cellular processes, particularly in the realms of neuroscience and cell biology. By enabling scientists to visualize changes in calcium ion concentrations, this method provides valuable insights into cellular activity and signaling pathways. It is an essential tool for understanding how cells communicate and respond to various stimuli, which is particularly crucial in studying neuronal dynamics and cardiac functions.
Definition and Importance
Calcium imaging can be defined as a technique used to observe the changes in intracellular calcium levels in real time. These changes are indicative of numerous cellular processes, making calcium ions a vital signaling molecule in a variety of biological contexts.
The importance of this technique lies in its ability to provide dynamic information about cellular responses under different conditions. For example, in neuroscience, calcium imaging helps researchers understand synaptic activity, neural network functions, and even behaviors associated with specific neuropathologies. The applicability extends beyond neuroscience, as it is also instrumental in studying muscle contractions, cellular responses in cancer, and interactions within immune cells.
Historical Development
The development of calcium imaging began in the 1980s, enabled by advancements in fluorescent indicators. The early work largely focused on single-cell measurements, utilizing fura-2, a well-known calcium indicator, which allowed scientists to measure intracellular calcium in live cells. Over the decades, the technology has evolved significantly.
With the introduction of genetically encoded calcium indicators (GECIs) in the late 1990s, the field saw a considerable transformation. These indicators allowed for more specific targeting within cells and facilitated long-term monitoring of cellular activities. Such advancements paved the way for high-throughput imaging techniques, enhancing the capacity to gather data across multiple cells simultaneously.
Fundamental Principles
The fundamental principle behind calcium imaging involves the detection of changes in fluorescence in response to varying calcium ion concentrations. When calcium binds to a fluorescent indicator, the indicator undergoes a conformational change that alters its fluorescent properties, most commonly leading to an increase in fluorescence intensity.
This can be measured using various microscopy techniques, whether it be wide-field fluorescence microscopy or two-photon microscopy. Each method has its advantages, with two-photon microscopy enabling deeper tissue imaging, which is critical for in vivo studies.
The analysis of collected data requires careful attention. The signals obtained can be influenced by factors such as the background fluorescence and the cellular environment, making data processing and interpretation key aspects of successful calcium imaging.
In essence, calcium imaging serves as a foundational technique for discerning complex biological processes. By understanding its definition, historical context, and fundamental principles, researchers can harness its potential and apply these insights effectively in diverse scientific inquiries.
Methodologies in Calcium Imaging
Calcium imaging plays a vital role in understanding cellular mechanisms in various biological contexts. It allows researchers to track calcium ion fluctuations within cells, typically signifying activity levels or physiological states. The methodologies employed in calcium imaging are crucial for ensuring accuracy, repeatability, and relevance of the data obtained. As science accelerates, several methodologies have emerged, providing researchers with advanced tools to obtain insights from their studies. This section delves into these methodologies, elucidating their significance and practical implications.
Imaging Techniques Overview
Different imaging techniques offer unique advantages and limitations. Understanding them is essential for selecting the appropriate method for specific experimental goals. Some of the most prominent techniques include:
- Two-Photon Microscopy: Ideal for deep tissue imaging and reducing phototoxicity.
- Wide-Field Fluorescence Microscopy: Useful for capturing rapid processes across larger areas.
- Miniaturized Imaging Systems: Emerging as powerful tools, especially in live animal studies.
Each technique has its own set of methods suited to various applications in research, thus impacting the quality of the data derived from imaging.
Two-Photon Microscopy
Two-photon microscopy utilizes longer wavelengths of light to achieve deeper penetration into tissues. This technique minimizes photodamage by exciting fluorescent indicators only at the focal point. Consequently, researchers can observe live tissues with minimal interference. The depth of imaging capability allows for investigations into brain networks or cellular interactions in intact systems.
This technique is particularly beneficial in neuroscience, where imaging deep brain structures is often crucial. Instruments that utilize this method are more complex and typically more expensive compared to their wide-field counterparts, yet they provide data that is often worth the investment.
Wide-Field Fluorescence Microscopy
Wide-field fluorescence microscopy allows for faster imaging over larger fields of view, enabling scientists to observe dynamic cellular events in real time. This method is advantageous for studies that require high-speed acquisition, such as monitoring calcium transients in excitable tissues.
However, the main limitation arises from the potential for increased background noise, leading to challenges in visualizing low-abundance indicators. Despite these limitations, wide-field microscopy remains a widely employed technique due to its accessibility and ability to capture rapid cellular processes efficiently.
Miniaturized Imaging Systems
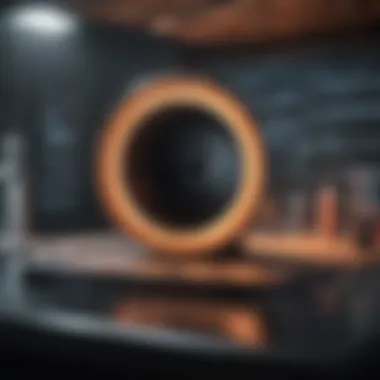
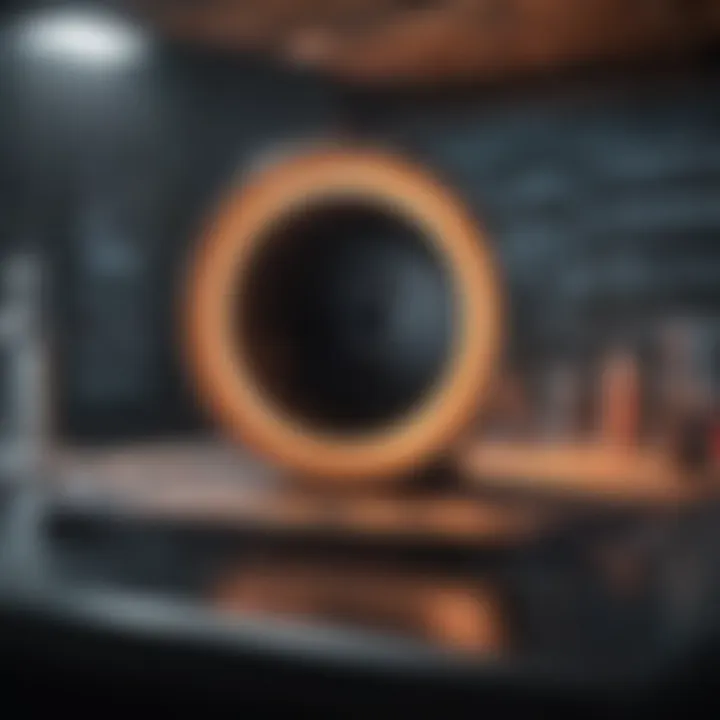
The advent of miniaturized imaging systems marks a significant stride in calcium imaging methodologies. These portable systems enable imaging in live animal models, thus bridging the gap between traditional methods and physiological relevance. Many efforts have gone into making these systems compact, allowing researchers to obtain data in freely moving organisms.
This methodology has opened new avenues for understanding dynamic networks in naturalistic settings. Given their flexibility, miniaturized systems are increasingly popular in behavioral neuroscience and pharmacology, where understanding real-time cellular responses in vivo is essential.
The choice of imaging technique must align with the specific research question to enhance data quality and interpretability.
Data Acquisition Techniques
Data acquisition techniques are central to the process of calcium imaging analysis. They serve as the foundation for capturing the dynamic changes in intracellular calcium levels, which are crucial for interpreting cellular activity. The choice of method directly influences the quality and reliability of the gathered data. Effective acquisition techniques not only enhance the sensitivity and specificity of the signals being measured but also streamline subsequent data processing and analysis.
Calcium Indicators
Calcium indicators play a vital role in the success of calcium imaging. These are molecules that exhibit changes in fluorescence intensity in response to shifts in calcium concentrations. Common indicators include Fura-2, Fluo-4, and GCaMP, which have different properties catering to specific requirements of a study.
The selection of a calcium indicator depends on several factors including:
- Calcium binding kinetics: Indicators must respond rapidly to calcium fluctuations for dynamic studies.
- Spectral properties: Excitation and emission wavelengths must align with available imaging technologies.
- Cell toxicity: A crucial consideration, as some indicators may adversely affect cell physiology or viability.
- Subcellular targeting: Certain studies might require indicators that localize to specific organelles.
The advancements in genetically encoded calcium indicators (GECIs) have broadened the scope for in vivo applications. GECIs like GCaMP allow for non-invasive, long-term monitoring of calcium levels in various models, increasing the potential for innovative applications in neuroscience.
Signal Processing
Once data is acquired, it undergoes rigorous signal processing, a critical step that enhances its clarity and usability. Processing techniques help mitigate noise and enhance the signal quality, enabling clearer interpretation of cellular dynamics. Several standard procedures in signal processing include:
- Background subtraction: Removing background noise is essential for accurate measurements of changes in fluorescence.
- Filtering: Using temporal filters can help eliminate high-frequency noise not associated with physiological activities.
- Normalization: This adjusts the signal to a common scale, making data comparison across experiments more feasible.
The success of signal processing directly impacts the integrity of the conclusions drawn from the calcium imaging data. Without meticulous handling during this stage, vital biological insights might be overlooked or misinterpreted.
3D Image Reconstruction
Three-dimensional image reconstruction allows researchers to view cellular activity in a more complex and realistic spatial context. This technique enables the visualization of how calcium levels vary not just across 2D planes, but also through the z-axis, creating a fuller picture of cellular dynamics. Although traditionally relying on advanced microscopy techniques, today’s algorithms facilitate impressive reconstructions from standard imaging data.
Key considerations in 3D reconstruction include:
- Dataset integration: Combining multiple 2D images captured from various depths can lead to comprehensive volumetric data.
- Volume rendering techniques: Different rendering methods may be employed, each with advantages in visualizing cellular structures and calcium dynamics.
- Software tools: Programs such as Imaris and Fiji offer users the capability to process and reconstruct 3D images effectively, making advanced imaging accessible to researchers.
The integration of 3D reconstruction into calcium imaging analysis significantly enriches our understanding of cellular processes and contributes to more accurate physiological models.
Applications of Calcium Imaging
Calcium imaging plays a critical role in various scientific disciplines, especially in understanding complex biological systems. Its widespread application stems from the ability to provide real-time insights into cellular and tissue-level activity. This technique allows researchers to study the dynamic behavior of calcium ions, which are vital for numerous physiological processes, making it an indispensable tool in modern research.
Neuroscience Research
In neuroscience, calcium imaging has transformed the way researchers investigate brain function. This technique enables the visualization of neuronal activity with remarkable spatial and temporal resolution. By using genetically encoded calcium indicators, scientists can monitor the activity of neurons in living organisms. This capability has provided significant insights into neural circuitry, synaptic plasticity, and the underlying mechanisms of learning and memory.
Moreover, calcium imaging allows for the examination of large populations of neurons simultaneously, leading to a more comprehensive understanding of brain networks. It is often utilized in studies of neurodevelopmental disorders, enabling researchers to explore aberrant calcium signaling in conditions such as autism spectrum disorders. This application underscores the importance of calcium imaging in advancing our knowledge of the brain's intricate functions.
Cardiovascular Studies
Calcium imaging is equally significant in cardiovascular research. It provides a pathway to investigate the role of calcium ions in cardiac myocyte function. Calcium dynamics are fundamental in the excitation-contraction coupling process, which is essential for heartbeats. Through this approach, researchers can analyze how calcium levels affect contractility, rhythm, and overall cardiac health.
Using calcium imaging techniques, abnormal calcium handling can be studied in various cardiovascular diseases, including heart failure and arrhythmias. This analysis can lead to improved diagnostic tools and therapeutic strategies targeting calcium signaling pathways, promising enhanced treatment options for patients suffering from cardiac conditions.
Cellular Dynamics in Cancer
In the field of cancer research, calcium imaging has emerged as a powerful tool to understand tumor biology. Cancer cells exhibit altered calcium signaling pathways, influencing cell proliferation, migration, and apoptosis. By applying calcium imaging, researchers can visualize abnormal calcium oscillations and signaling in tumor cells.
This technique provides crucial information on how cancer cells communicate and respond to their microenvironment, shedding light on metastatic potential and therapeutic resistance. Furthermore, calcium imaging can aid in the evaluation of drug responses, allowing for the development of more effective cancer treatments.
In summary, the applications of calcium imaging are vast and invaluable. It offers critical insights into cellular mechanisms across various disciplines, facilitating breakthroughs in neuroscience, cardiovascular health, and cancer research. As this method continues to evolve, its potential for enhancing our understanding of complex biological systems is bound to expand.
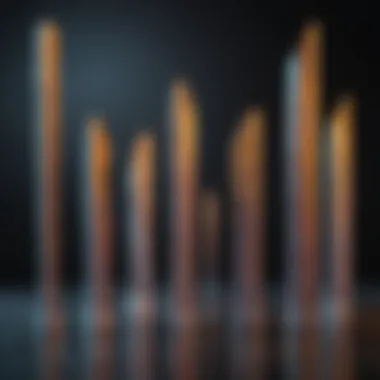
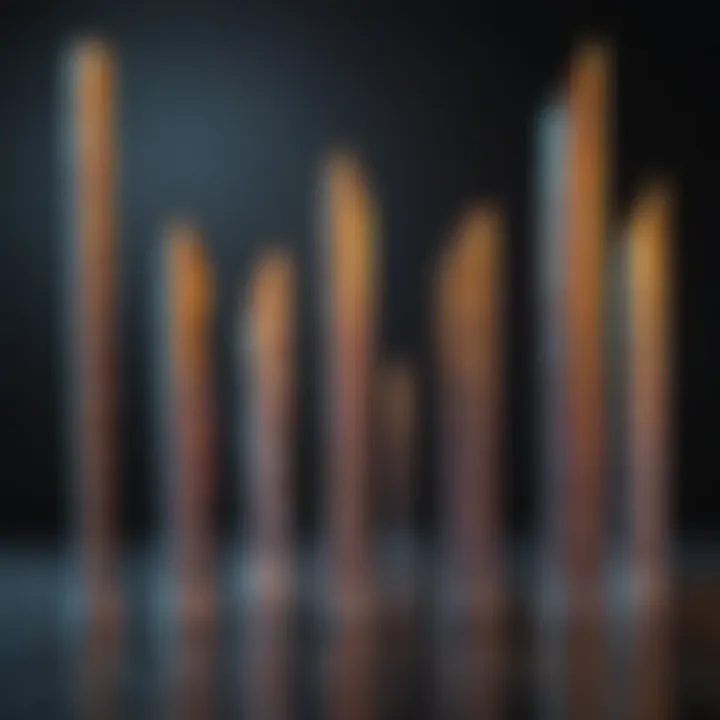
Challenges in Calcium Imaging
The field of calcium imaging analysis faces several significant challenges that can impact the integrity and accuracy of the data collected. Addressing these challenges is crucial for researchers aiming to utilize this technique effectively. Understanding the limitations and intricacies involved in calcium imaging is vital for informed decision-making in experimental design and data interpretation.
Signal-to-Noise Ratio
The signal-to-noise ratio (SNR) is a critical factor in calcium imaging. A high SNR indicates that the signal from calcium indicators is strong relative to background noise, allowing for better detection of neuronal activity. Low SNR can lead to difficulties in distinguishing actual calcium signals from noise generated by various sources, such as electronic interference or spontaneous fluorescence.
Researchers often employ advanced filtering techniques or optimize imaging conditions to improve SNR. For example:
- Use of higher sensitive detectors: This can enhance the collection of faint signals.
- Signal averaging: Combining multiple frames can reduce the impact of random noise.
- Image denoising algorithms: These can help in removing unwanted noise while preserving true signals.
Spatial and Temporal Resolution
Spatial and temporal resolution are also pivotal in determining the quality of calcium imaging data. Spatial resolution refers to the ability to resolve details in the image, which is crucial for discerning between closely located neuronal populations. Low spatial resolution can result in blurred images that obscure the dynamics of cellular interactions.
Temporal resolution, on the other hand, relates to the frequency of image acquisition. If temporal resolution is insufficient, fast calcium transients may be missed, leading to underestimation of neuronal activity levels. Balancing these two types of resolution often requires trade-offs. Increasing spatial resolution usually demands longer exposure times, which can compromise temporal resolution. Techniques like high-speed cameras and advanced algorithms play a role in mitigating this challenge by offering a balance between these two aspects.
Data Interpretation Challenges
Data interpretation in calcium imaging involves complex analytical methods that can introduce additional layers of difficulty. The fluctuations in calcium signals can be influenced by a range of biological processes, making it challenging to ascertain the exact source of the signals detected. Moreover, the dynamics of calcium signaling can vary across cell types and conditions, further complicating the analysis.
Researchers must be cautious while interpreting the data. Common challenges include:
- Overfitting models: Researchers can inadvertently tailor models too closely to existing datasets, which may not generalize well to new data.
- Bias in selection of analysis methods: Relying too heavily on specific analytical tools without exploring alternatives can limit the understanding of the underlying biology.
- Integration with other data types: Combining calcium imaging data with electrophysiological data or behavioral observations requires a rigorous approach to ensure that conclusions made are robust and reliable.
Overall, tackling the challenges posed by signal-to-noise ratio, spatial and temporal resolution, and data interpretation is essential for advancing the field of calcium imaging analysis. By recognizing these obstacles and employing appropriate strategies to mitigate them, researchers can significantly enhance the reliability of their findings.
Technological Advancements
Technological advancements play a crucial role in the field of calcium imaging analysis. As researchers strive for enhanced accuracy and efficiency, new developments in technology continually reshape the landscape. These advancements lead to significant improvements in data collection methods, facilitate more complex experimental designs, and enhance the interpretability of results. Here, we explore some of the key innovations that are transforming calcium imaging techniques and their applications.
Development of New Fluorescent Indicators
The evolution of fluorescent indicators marks a significant milestone in calcium imaging. New indicators are developed to be more sensitive and specific to calcium concentrations. Commonly used indicators like GCaMP and Cal-520 have made it possible to capture real-time fluctuations in calcium levels with unparalleled clarity.
Advancements also include the optimization of these indicators for various applications. For instance, newer variants can operate effectively in different cellular environments, offering flexibility in experimental setups.
- Enhanced brightness and photostability allow for longer imaging sessions without significant degradation of the signal.
- Indicators with faster kinetics enable researchers to monitor rapid calcium transients that occur in neurons during action potentials.
These innovations have led to a more detailed understanding of cellular dynamics that was not previously accessible.
Integration with Optogenetics
Optogenetics and calcium imaging are increasingly being used together, creating a powerful combination for the study of neuronal circuits. The integration of optogenetics allows for precise control over neuronal activity while simultaneously measuring calcium signals. This dual approach facilitates a deeper understanding of how neurons communicate and respond to stimuli.
Benefits of this integration include:
- Temporal Precision: Researchers can activate or inhibit specific populations of neurons at precise time intervals, allowing for the correlation of electrical activity with calcium signaling.
- Functional Mapping: It provides insights into causal relationships in neural networks, shedding light on both normal physiological functions and pathological conditions.
The combined use of these technologies opens new avenues for understanding complex behaviors and their underlying neurobiological mechanisms.
Autonomously Operated Imaging Systems
The development of autonomously operated imaging systems represents another leap forward in this field. These systems can be programmed to perform imaging tasks with minimal human intervention, reflecting advances in automation and robotics.
Such systems offer several advantages:
- Increased Throughput: By automating the imaging process, researchers can collect data across multiple samples simultaneously, thus enhancing efficiency.
- Consistency: Automated systems reduce human error, ensuring that data acquisition is uniform across experiments, which enhances reproducibility.
- Real-Time Analysis: Many modern imaging systems come equipped with real-time data processing capabilities, allowing scientists to analyze results as they are generated.
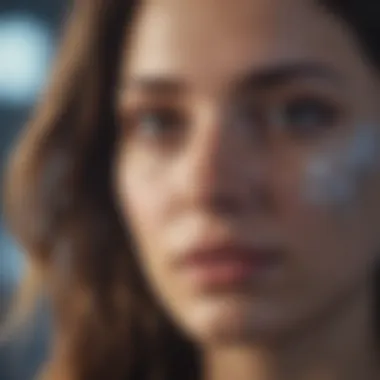
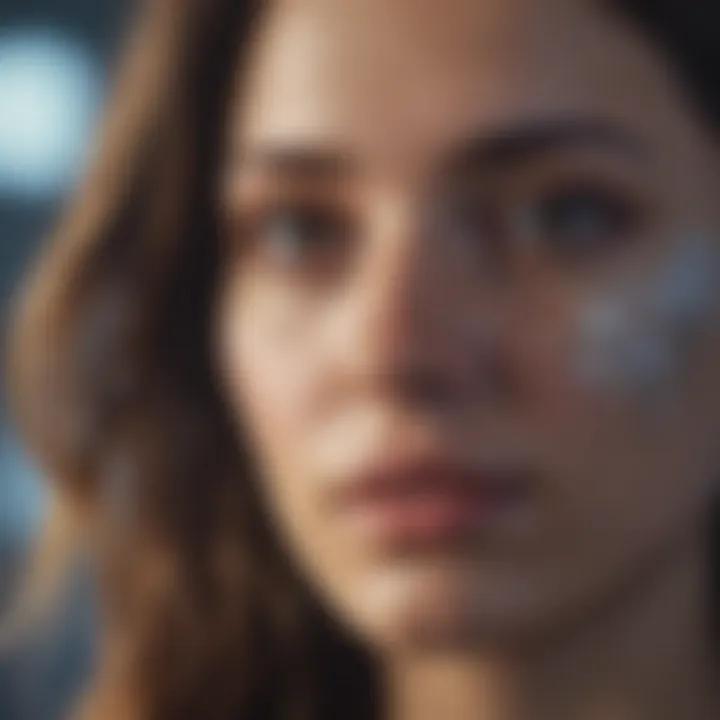
In summary, technological advancements in calcium imaging analysis are pivotal to the progress of research in neurosciences and cell biology. From developing new fluorescent indicators to integrating with optogenetics, and implementing autonomously operated systems, these innovations not only boost the quality of data but also expand the range of applications.
"Technology and innovative thinking are essential to unlock new possibilities in calcium imaging, ultimately leading to breakthroughs in our understanding of living systems."
Such progress paves the way for future explorations and applications in understanding cellular behavior across different conditions.
Future Perspectives
The field of calcium imaging is evolving rapidly, driving significant advancements in both research and practical applications. As scientists deepen their understanding of calcium signaling in various biological systems, several promising future perspectives emerge. These trends not only aim to enhance the precision and efficacy of existing methodologies but also address pressing challenges that researchers face today.
Emerging Research Trends
Recent studies in calcium imaging reveal a focus on uncovering the intricate mechanisms of signal transduction. More specifically, researchers are exploring the interaction between calcium ions and various cellular pathways. This is crucial for understanding diverse biological processes, such as synaptic plasticity in neurons or the regulation of cardiac rhythm.
Another emerging trend is the development of hybrid imaging techniques. For instance, the combination of calcium imaging with advanced microscopies like super-resolution microscopy allows for a more localized analysis of cellular behavior. This approach is proving beneficial in elucidating complex network dynamics in live cells. When researchers utilize these integrated methods, they gain deeper insights into how cells respond to stimuli in real time.
Moreover, artificial intelligence and machine learning are becoming pivotal in analyzing vast datasets generated from calcium imaging. Automated algorithms can now sift through and interpret patterns of calcium influx, potentially unveiling previously unrecognized relationships between cellular activity and physiological responses.
Potential in Disease Modeling
Calcium imaging holds significant potential in disease modeling, particularly in neurodegenerative disorders and cancer research. One key benefit is the ability to observe real-time dynamic processes in cells that may be altered in disease states. For example, studying calcium signaling in neurons allows researchers to identify early pathological changes that characterize diseases like Alzheimer’s or Parkinson’s.
Through calcium imaging, scientists can model disease progression by replicating cellular environments affected by such disorders. This facilitates the testing of pharmaceutical interventions in a controlled setting, offering a preview of therapeutic efficacy before human trials.
Furthermore, calcium imaging technology can support personalized medicine. By analyzing a patient’s unique cellular responses, clinicians can better tailor treatment strategies to individual needs, highlighting a significant advancement in the realm of patient care.
In summary, the future of calcium imaging analysis is bright, with emerging trends paving the way for new research opportunities. The techniques' applicability in disease modeling can enhance understanding and treatment of complex conditions and ultimately favor advances in health and well-being.
"As calcium imaging technologies advance, they pave new pathways in understanding cellular mechanisms and reimagining treatment paradigms across disciplines."
Ethical Considerations
Ethical considerations play a crucial role in the domain of calcium imaging analysis. As this technique is often applied in research that involves living organisms, ethical aspects must not be overlooked. Researchers must ensure they conduct their studies with the highest regard for the welfare of their subjects. This section examines the significance of ethical practices in calcium imaging research, emphasizing two main areas: animal welfare and data privacy.
Animal Welfare in Research
Animal welfare is a primary concern in scientific research, particularly in studies that require in vivo calcium imaging. The use of animal models is integral to understanding complex biological processes and diseases, yet it must be balanced with ethical obligations to minimize harm.
- Regulatory Framework: There are strict regulations governing the use of animals in research, such as the Animal Welfare Act in the United States and similar laws in other countries. These laws mandate that researchers must apply alternatives wherever possible and ensure humane treatment throughout the studies.
- Reduction and Refinement: Researchers are encouraged to adopt the 3Rs principle: Replacement, Reduction, and Refinement. This means finding alternatives to animal studies when feasible, minimizing the number of animals used, and refining techniques to reduce suffering and improve animal welfare outcomes.
- Right Procedures: Calicum imaging studies must involve appropriate anesthesia for any invasive procedures, minimizing stress and pain throughout the study. Ensuring that researchers are trained in animal handling and welfare improves the ethical conduct of research.
In summary, the commitment to animal welfare underscores the responsibility that researchers hold. Failure to adhere to these principles can have far-reaching implications, not only for the subjects involved but also for the integrity of the scientific community.
Data Privacy and Compliance
Data privacy and compliance are becoming increasingly important in research involving calcium imaging, particularly as data sets grow in size and complexity. The ethical handling of data related to human subjects, which may be collected indirectly through animal models, is paramount.
- Informed Consent: For studies involving human data, obtaining informed consent is crucial. This ensures that individuals are aware of how their data will be used and the potential risks involved.
- Anonymization of Data: It is essential to anonymize any sensitive information to protect individual privacy. Researchers must ensure that any identifiable information is removed from datasets before analysis.
- Compliance with Legal Frameworks: Researchers must adhere to applicable laws such as the General Data Protection Regulation (GDPR) in the European Union or the Health Insurance Portability and Accountability Act (HIPAA) in the United States, which set strict guidelines on data collection, storage, and sharing.
Overall, the ethical dimensions surrounding data privacy require a comprehensive understanding of current laws and practices. Compliance ensures both the protection of individual rights and the credibility of research findings.
In sum, ethical considerations in calcium imaging involve safeguarding animal welfare and ensuring data privacy. Maintaining these ethical standards is essential for the scientific community, researchers' reputations, and the welfare of society overall. By addressing these matters, we foster an environment that values ethical conduct while advancing scientific knowledge.
Culmination
The importance of the conclusion in this article lies in its ability to summarize the complex journey through calcium imaging analysis. It brings together the various elements discussed in the previous sections, highlighting the significance of this technique in contemporary scientific research. By reinforcing the core principles and methodologies explored, the conclusion serves as a reflective pause for readers to grasp how the intricacies of calcium imaging have reshaped our understanding of cellular processes.
Recap of Key Points
In revisiting the critical aspects of calcium imaging, key points include:
- Definition and Importance: Calcium imaging is a vital tool for mapping the activity of cells, crucial in fields like neuroscience and cell biology.
- Methodologies: Different imaging techniques, such as two-photon microscopy and wide-field fluorescence microscopy, provide varied approaches to data acquisition.
- Applications: It has diverse applications ranging from studying neural circuits to understanding cardiovascular systems.
- Challenges: The field faces challenges regarding signal-to-noise ratio and spatial resolution, which impacts data quality.
- Technological Advancements: New fluorescent indicators and integration with optogenetics have advanced both the methodologies and potential applications.
The takeaway from these points is clear: calcium imaging analysis is not just a technique but a lens through which complex biological processes can be understood and interpreted.
Final Thoughts on Calcium Imaging Analysis
The integration of ethical considerations, particularly regarding animal welfare and data privacy, remains paramount as the field evolves. Overall, the body of work around calcium imaging emphasizes its crucial role in advancing our understanding of biology, ultimately improving health outcomes and scientific knowledge.